Mechanisms of activation, inhibition and specificity: crystal structures of the NMDA receptor NR1 ligand-binding core
- PMID: 12805203
- PMCID: PMC162155
- DOI: 10.1093/emboj/cdg303
Mechanisms of activation, inhibition and specificity: crystal structures of the NMDA receptor NR1 ligand-binding core
Abstract
Excitatory neurotransmission mediated by the N-methyl-D-aspartate subtype of ionotropic glutamate receptors is fundamental to the development and function of the mammalian central nervous system. NMDA receptors require both glycine and glutamate for activation with NR1 and NR2 forming glycine and glutamate sites, respectively. Mechanisms to describe agonist and antagonist binding, and activation and desensitization of NMDA receptors have been hampered by the lack of high-resolution structures. Here, we describe the cocrystal structures of the NR1 S1S2 ligand-binding core with the agonists glycine and D-serine (DS), the partial agonist D-cycloserine (DCS) and the antagonist 5,7-dichlorokynurenic acid (DCKA). The cleft of the S1S2 'clamshell' is open in the presence of the antagonist DCKA and closed in the glycine, DS and DCS complexes. In addition, the NR1 S1S2 structure reveals the fold and interactions of loop 1, a cysteine-rich region implicated in intersubunit allostery.
Figures
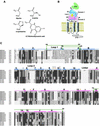
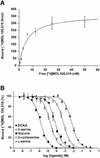
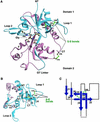
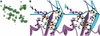
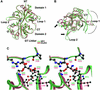
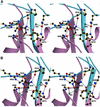
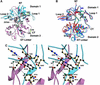
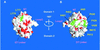
Similar articles
-
Model structures of the N-methyl-D-aspartate receptor subunit NR1 explain the molecular recognition of agonist and antagonist ligands.J Struct Biol. 2004 Mar;145(3):205-15. doi: 10.1016/j.jsb.2003.10.016. J Struct Biol. 2004. PMID: 14960371
-
Evolutionary trace analysis of ionotropic glutamate receptor sequences and modeling the interactions of agonists with different NMDA receptor subunits.J Mol Model. 2004 Dec;10(5-6):305-16. doi: 10.1007/s00894-004-0196-7. Epub 2004 Oct 22. J Mol Model. 2004. PMID: 15597199
-
Structural consequences of D481N/K483Q mutation at glycine binding site of NMDA ionotropic glutamate receptors: a molecular dynamics study.J Biomol Struct Dyn. 2005 Feb;22(4):399-410. doi: 10.1080/07391102.2005.10507012. J Biomol Struct Dyn. 2005. PMID: 15588104
-
Influence of the NR3A subunit on NMDA receptor functions.Prog Neurobiol. 2010 May;91(1):23-37. doi: 10.1016/j.pneurobio.2010.01.004. Epub 2010 Jan 25. Prog Neurobiol. 2010. PMID: 20097255 Free PMC article. Review.
-
Shuffling the deck anew: how NR3 tweaks NMDA receptor function.Mol Neurobiol. 2008 Aug;38(1):16-26. doi: 10.1007/s12035-008-8029-9. Epub 2008 Jul 25. Mol Neurobiol. 2008. PMID: 18654865 Review.
Cited by
-
Allosteric signaling and dynamics of the clamshell-like NMDA receptor GluN1 N-terminal domain.Nat Struct Mol Biol. 2013 Apr;20(4):477-85. doi: 10.1038/nsmb.2522. Epub 2013 Mar 3. Nat Struct Mol Biol. 2013. PMID: 23454977
-
Glycine-dependent activation of NMDA receptors.J Gen Physiol. 2015 Jun;145(6):513-27. doi: 10.1085/jgp.201411302. Epub 2015 May 11. J Gen Physiol. 2015. PMID: 25964432 Free PMC article.
-
An NMDA receptor gating mechanism developed from MD simulations reveals molecular details underlying subunit-specific contributions.Biophys J. 2013 May 21;104(10):2170-81. doi: 10.1016/j.bpj.2013.04.013. Biophys J. 2013. PMID: 23708357 Free PMC article.
-
Conformational dynamics of the ligand-binding domain of inward rectifier K channels as revealed by molecular dynamics simulations: toward an understanding of Kir channel gating.Biophys J. 2005 May;88(5):3310-20. doi: 10.1529/biophysj.104.052019. Epub 2005 Mar 4. Biophys J. 2005. PMID: 15749783 Free PMC article.
-
Antidepressant interactions with the NMDA NR1-1b subunit.J Biophys. 2008;2008:474205. doi: 10.1155/2008/474205. Epub 2008 Jun 5. J Biophys. 2008. PMID: 20107576 Free PMC article.
References
-
- Aizenman E., Lipton,S.A. and Loring,R.H. (1989) Selective modulation of NMDA responses by reduction and oxidation. Neuron, 2, 1257–1263. - PubMed
-
- Armstrong N. and Gouaux,E. (2000) Mechanisms for activation and antagonism of an AMPA-sensitive glutamate receptor: crystal structures of the GluR2 ligand binding core. Neuron, 28, 165–181. - PubMed
-
- Armstrong N., Sun,Y., Chen,G.-Q. and Gouaux,E. (1998) Structure of a glutamate receptor ligand binding core in complex with kainate. Nature, 395, 913–917. - PubMed
-
- Brunger A.T. et al. (1998) Crystallography and NMR system: A new software suite for macromolecular structure determination. Acta Crystallogr. D, 54, 905–921. - PubMed
-
- CCP4 (1994) The CCP4 suite: programs for protein crystallography. Acta Crystallogr.D, 50, 760–763. - PubMed
Publication types
MeSH terms
Substances
Associated data
- Actions
- Actions
- Actions
- Actions
LinkOut - more resources
Full Text Sources
Molecular Biology Databases