Characterization of the WAVE1 knock-out mouse: implications for CNS development
- PMID: 12716942
- PMCID: PMC6742295
- DOI: 10.1523/JNEUROSCI.23-08-03343.2003
Characterization of the WAVE1 knock-out mouse: implications for CNS development
Abstract
Developing neurons must respond to a wide range of extracellular signals during the process of brain morphogenesis. One mechanism through which immature neurons respond to such signals is by altering cellular actin dynamics. A recently discovered link between extracellular signaling events and the actin cytoskeleton is the WASP/WAVE (Wiscott-Aldrich Syndrome protein/WASP-family verprolin-homologous protein) family of proteins. Through a direct interaction with the Arp2/3 (actin-related protein) complex, this family functions to regulate the actin cytoskeleton by mediating signals from cdc42 as well as other small GTPases. To evaluate the role of WASP/WAVE proteins in the process of neuronal morphogenesis, we used a retroviral gene trap to generate a line of mice bearing a disruption in the WAVE1 gene. Using a heterologous reporter gene, we found that WAVE1 expression becomes increasingly restricted to the CNS over the course of development. Homozygous disruption of the WAVE1 gene results in postnatal lethality. In addition, these animals have severe limb weakness, a resting tremor, and notable neuroanatomical malformations without overt histopathology of peripheral organs. We did not detect any alterations in neuronal morphology in vivo or the ability of embryonic neurons to form processes in vitro. Our data indicate that WAVE1, although important for the general development of the CNS, is not essential for the formation and extension of neuritic processes.
Figures
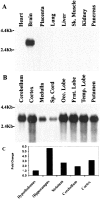
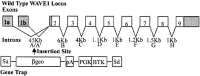
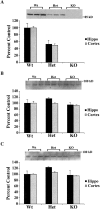
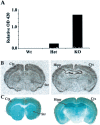
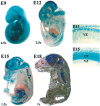
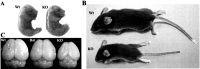
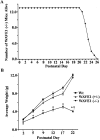
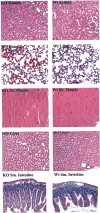
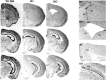
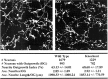
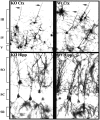
Similar articles
-
Characterization and expression analyses of the mouse Wiskott-Aldrich syndrome protein (WASP) family member Wave1/Scar.Gene. 2002 May 15;290(1-2):131-40. doi: 10.1016/s0378-1119(02)00560-7. Gene. 2002. PMID: 12062808
-
Two verprolin homology domains increase the Arp2/3 complex-mediated actin polymerization activities of N-WASP and WAVE1 C-terminal regions.Biochem Biophys Res Commun. 2002 Sep 20;297(2):214-9. doi: 10.1016/s0006-291x(02)02137-x. Biochem Biophys Res Commun. 2002. PMID: 12237104
-
WAVE1 is required for oligodendrocyte morphogenesis and normal CNS myelination.J Neurosci. 2006 May 24;26(21):5849-59. doi: 10.1523/JNEUROSCI.4921-05.2006. J Neurosci. 2006. PMID: 16723544 Free PMC article.
-
The Role of WAVE2 Signaling in Cancer.Biomedicines. 2021 Sep 14;9(9):1217. doi: 10.3390/biomedicines9091217. Biomedicines. 2021. PMID: 34572403 Free PMC article. Review.
-
The WASP-WAVE protein network: connecting the membrane to the cytoskeleton.Nat Rev Mol Cell Biol. 2007 Jan;8(1):37-48. doi: 10.1038/nrm2069. Nat Rev Mol Cell Biol. 2007. PMID: 17183359 Review.
Cited by
-
Mitochondrial a Kinase Anchor Proteins in Cardiovascular Health and Disease: A Review Article on Behalf of the Working Group on Cellular and Molecular Biology of the Heart of the Italian Society of Cardiology.Int J Mol Sci. 2022 Jul 12;23(14):7691. doi: 10.3390/ijms23147691. Int J Mol Sci. 2022. PMID: 35887048 Free PMC article. Review.
-
Rho GTPase Regulators and Effectors in Autism Spectrum Disorders: Animal Models and Insights for Therapeutics.Cells. 2020 Mar 31;9(4):835. doi: 10.3390/cells9040835. Cells. 2020. PMID: 32244264 Free PMC article. Review.
-
The intrinsically disordered cytoplasmic tail of a dendrite branching receptor uses two distinct mechanisms to regulate the actin cytoskeleton.Elife. 2023 Aug 9;12:e88492. doi: 10.7554/eLife.88492. Elife. 2023. PMID: 37555826 Free PMC article.
-
RHOA and mDia1 Promotes Apoptosis of Breast Cancer Cells Via a High Dose of Doxorubicin Treatment.Open Life Sci. 2019 Dec 31;14:619-627. doi: 10.1515/biol-2019-0070. eCollection 2019 Jan. Open Life Sci. 2019. PMID: 33817200 Free PMC article.
-
WAVE3, an actin remodeling protein, is regulated by the metastasis suppressor microRNA, miR-31, during the invasion-metastasis cascade.Int J Cancer. 2011 Sep 15;129(6):1331-43. doi: 10.1002/ijc.25793. Epub 2011 Mar 22. Int J Cancer. 2011. PMID: 21105030 Free PMC article.
References
-
- Banzai Y, Miki H, Yamaguchi H, Takenawa T. Essential role of neural Wiskott-Aldrich syndrome protein in neurite extension in PC12 cells and rat hippocampal primary culture cells. J Biol Chem. 2000;275:11987–11992. - PubMed
-
- Butt E, Abel K, Krieger M, Palm D, Hoppe V, Hoppe J, Walter U. cAMP- and cGMP-dependent protein kinase phosphorylation sites of the focal adhesion vasodilator-stimulated phosphoprotein (VASP) in vitro and in intact human platelets. J Biol Chem. 1994;269:14509–14517. - PubMed
-
- Derry JM, Ochs HD, Francke U. Isolation of a novel gene mutated in Wiskott-Aldrich syndrome. Cell. 1994;78:635–644. - PubMed
-
- Gertler FB, Niebuhr K, Reinhard M, Wehland J, Soriano P. Mena, a relative of VASP and Drosophila Enabled, is implicated in the control of microfilament dynamics. Cell. 1996;87:227–239. - PubMed
MeSH terms
Substances
LinkOut - more resources
Full Text Sources
Medical
Molecular Biology Databases
Miscellaneous