Stop codon selection in eukaryotic translation termination: comparison of the discriminating potential between human and ciliate eRF1s
- PMID: 12660170
- PMCID: PMC152891
- DOI: 10.1093/emboj/cdg146
Stop codon selection in eukaryotic translation termination: comparison of the discriminating potential between human and ciliate eRF1s
Abstract
During eukaryotic translation termination, eRF1 responds to three stop codons. However, in ciliates with variant genetic codes, only one or two codons function as a stop signal. To localize the region of ciliate eRF1 implicated in stop codon discrimination, we have constructed ciliate-human hybrid eRF1s by swapping regions of human eRF1 for the equivalent region of ciliate Euplotes eRF1. We have examined the formation of a cross-link between recombinant eRF1s and mRNA analogs containing the photoactivable 4-thiouridine (s(4)U) at the first position of stop and control sense codons. With human eRF1, this cross-link can be detected only when either stop or UGG codons are located in the ribosomal A site. Here we show that the cross-link of the Euplotes-human hybrid eRF1 is restricted to mRNAs containing UAG and UAA codons, and that the entire N-terminal domain of Euplotes eRF1 is involved in discriminating against UGA and UGG. On the basis of these results, we discuss the steps of the selection process that determine the accuracy of stop codon recognition in eukaryotes.
Figures
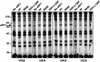
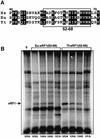
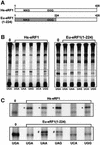
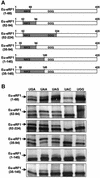
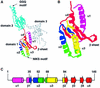
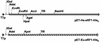
Similar articles
-
Different modes of stop codon restriction by the Stylonychia and Paramecium eRF1 translation termination factors.Proc Natl Acad Sci U S A. 2007 Jun 26;104(26):10824-9. doi: 10.1073/pnas.0703887104. Epub 2007 Jun 15. Proc Natl Acad Sci U S A. 2007. PMID: 17573528 Free PMC article.
-
[How translation termination factor eRF1 Euplotes does not recognise UGA stop codon].Mol Biol (Mosk). 2007 Nov-Dec;41(6):1014-22. Mol Biol (Mosk). 2007. PMID: 18318120 Russian.
-
A single amino acid change of translation termination factor eRF1 switches between bipotent and omnipotent stop-codon specificity.Nucleic Acids Res. 2011 Jan;39(2):599-608. doi: 10.1093/nar/gkq759. Epub 2010 Sep 21. Nucleic Acids Res. 2011. PMID: 20860996 Free PMC article.
-
[Molecular mechanism of stop codon recognition by eRF1: a wobble hypothesis for peptide anticodons].Tanpakushitsu Kakusan Koso. 2001 Dec;46(15):2163-70. Tanpakushitsu Kakusan Koso. 2001. PMID: 11762076 Review. Japanese. No abstract available.
-
Termination of translation in eukaryotes.Biochem Cell Biol. 1995 Nov-Dec;73(11-12):1079-86. doi: 10.1139/o95-116. Biochem Cell Biol. 1995. PMID: 8722024 Review.
Cited by
-
Two-step model of stop codon recognition by eukaryotic release factor eRF1.Nucleic Acids Res. 2013 Apr;41(8):4573-86. doi: 10.1093/nar/gkt113. Epub 2013 Feb 23. Nucleic Acids Res. 2013. PMID: 23435318 Free PMC article.
-
Translation termination in pyrrolysine-utilizing archaea.FEBS Lett. 2009 Nov 3;583(21):3455-60. doi: 10.1016/j.febslet.2009.09.044. Epub 2009 Sep 29. FEBS Lett. 2009. PMID: 19796638 Free PMC article.
-
Therapeutics based on stop codon readthrough.Annu Rev Genomics Hum Genet. 2014;15:371-94. doi: 10.1146/annurev-genom-091212-153527. Epub 2014 Apr 18. Annu Rev Genomics Hum Genet. 2014. PMID: 24773318 Free PMC article. Review.
-
New insights into stop codon recognition by eRF1.Nucleic Acids Res. 2015 Mar 31;43(6):3298-308. doi: 10.1093/nar/gkv154. Epub 2015 Mar 3. Nucleic Acids Res. 2015. PMID: 25735746 Free PMC article.
-
Different modes of stop codon restriction by the Stylonychia and Paramecium eRF1 translation termination factors.Proc Natl Acad Sci U S A. 2007 Jun 26;104(26):10824-9. doi: 10.1073/pnas.0703887104. Epub 2007 Jun 15. Proc Natl Acad Sci U S A. 2007. PMID: 17573528 Free PMC article.
References
-
- Brown C.M. and Tate,W.P. (1994) Direct recognition of mRNA stop signals by Escherichia coli polypeptide chain release factor two. J. Biol. Chem., 269, 33164–33170. - PubMed
-
- Caron F. and Meyer,E. (1985) Does Paramecium primaurelia use a different genetic code in its macronucleus? Nature, 314, 185–188. - PubMed
-
- Chavatte L., Frolova,L., Kisselev,L. and Favre,A. (2001) The polypeptide chain release factor eRF1 specifically contacts the s4UGA stop codon located in the A site of eukaryotic ribosomes. Eur. J. Biochem., 268, 2896–2904. - PubMed
Publication types
MeSH terms
Substances
LinkOut - more resources
Full Text Sources