Cells lying on a bed of microneedles: an approach to isolate mechanical force
- PMID: 12552122
- PMCID: PMC149857
- DOI: 10.1073/pnas.0235407100
Cells lying on a bed of microneedles: an approach to isolate mechanical force
Abstract
We describe an approach to manipulate and measure mechanical interactions between cells and their underlying substrates by using microfabricated arrays of elastomeric, microneedle-like posts. By controlling the geometry of the posts, we varied the compliance of the substrate while holding other surface properties constant. Cells attached to, spread across, and deflected multiple posts. The deflections of the posts occurred independently of neighboring posts and, therefore, directly reported the subcellular distribution of traction forces. We report two classes of force-supporting adhesions that exhibit distinct force-size relationships. Force increased with size of adhesions for adhesions larger than 1 microm(2), whereas no such correlation existed for smaller adhesions. By controlling cell adhesion on these micromechanical sensors, we showed that cell morphology regulates the magnitude of traction force generated by cells. Cells that were prevented from spreading and flattening against the substrate did not contract in response to stimulation by serum or lysophosphatidic acid, whereas spread cells did. Contractility in the unspread cells was rescued by expression of constitutively active RhoA. Together, these findings demonstrate a coordination of biochemical and mechanical signals to regulate cell adhesion and mechanics, and they introduce the use of arrays of mechanically isolated sensors to manipulate and measure the mechanical interactions of cells.
Figures
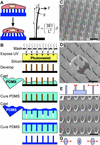
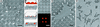
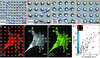
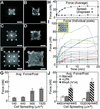
Comment in
-
Mechanosensation through integrins: cells act locally but think globally.Proc Natl Acad Sci U S A. 2003 Feb 18;100(4):1472-4. doi: 10.1073/pnas.0530201100. Epub 2003 Feb 10. Proc Natl Acad Sci U S A. 2003. PMID: 12578965 Free PMC article. No abstract available.
Similar articles
-
Magnetic microposts as an approach to apply forces to living cells.Proc Natl Acad Sci U S A. 2007 Sep 11;104(37):14553-8. doi: 10.1073/pnas.0611613104. Epub 2007 Sep 5. Proc Natl Acad Sci U S A. 2007. PMID: 17804810 Free PMC article.
-
The regulation of traction force in relation to cell shape and focal adhesions.Biomaterials. 2011 Mar;32(8):2043-51. doi: 10.1016/j.biomaterials.2010.11.044. Epub 2010 Dec 15. Biomaterials. 2011. PMID: 21163521 Free PMC article.
-
Traction forces exerted by epithelial cell sheets.J Phys Condens Matter. 2010 May 19;22(19):194119. doi: 10.1088/0953-8984/22/19/194119. Epub 2010 Apr 26. J Phys Condens Matter. 2010. PMID: 21386442
-
Mechanical cues direct focal adhesion dynamics.Prog Mol Biol Transl Sci. 2014;126:103-34. doi: 10.1016/B978-0-12-394624-9.00005-1. Prog Mol Biol Transl Sci. 2014. PMID: 25081616 Review.
-
Biochemistry and biomechanics of cell motility.Annu Rev Biomed Eng. 2005;7:105-50. doi: 10.1146/annurev.bioeng.7.060804.100340. Annu Rev Biomed Eng. 2005. PMID: 16004568 Review.
Cited by
-
Mechanobiology in lung epithelial cells: measurements, perturbations, and responses.Compr Physiol. 2012 Jan;2(1):1-29. doi: 10.1002/cphy.c100090. Compr Physiol. 2012. PMID: 23728969 Free PMC article. Review.
-
Cell spreading area regulates clathrin-coated pit dynamics on micropatterned substrate.Integr Biol (Camb). 2015 Sep;7(9):1033-43. doi: 10.1039/c5ib00111k. Epub 2015 Jul 24. Integr Biol (Camb). 2015. PMID: 26205141 Free PMC article.
-
Optically monitored drug delivery patch based on porous silicon and polymer microneedles.Biomed Opt Express. 2016 Apr 4;7(5):1645-55. doi: 10.1364/BOE.7.001645. eCollection 2016 May 1. Biomed Opt Express. 2016. PMID: 27231611 Free PMC article.
-
Model-based traction force microscopy reveals differential tension in cellular actin bundles.PLoS Comput Biol. 2015 Mar 6;11(3):e1004076. doi: 10.1371/journal.pcbi.1004076. eCollection 2015 Mar. PLoS Comput Biol. 2015. PMID: 25748431 Free PMC article.
-
Nucleocytoplasmic transport senses mechanical forces independently of cell density in cell monolayers.J Cell Sci. 2024 Sep 1;137(17):jcs262363. doi: 10.1242/jcs.262363. Epub 2024 Sep 9. J Cell Sci. 2024. PMID: 39120491 Free PMC article.
References
-
- Couchman J R, Rees D A. Cell Biol Int Rep. 1979;3:431–439. - PubMed
-
- Miyamoto S, Akiyama S K, Yamada K M. Science. 1995;267:883–885. - PubMed
-
- Yamada K M, Olden K. Nature. 1978;275:179–184. - PubMed
-
- Folkman J, Moscona A. Nature. 1978;273:345–349. - PubMed
-
- Harris A K, Wild P, Stopak D. Science. 1980;208:177–179. - PubMed
Publication types
MeSH terms
Grants and funding
LinkOut - more resources
Full Text Sources
Other Literature Sources