The role of neurotrophins in neurotransmitter release
- PMID: 12467374
- PMCID: PMC2810653
- DOI: 10.1177/1073858402238511
The role of neurotrophins in neurotransmitter release
Abstract
The neurotrophins (NTs) have recently been shown to elicit pronounced effects on quantal neurotransmitter release at both central and peripheral nervous system synapses. Due to their activity-dependent release, as well as the subcellular localization of both protein and receptor, NTs are ideally suited to modify the strength of neuronal connections by "fine-tuning" synaptic activity through direct actions at presynaptic terminals. Here, using BDNF as a prototypical example, the authors provide an update of recent evidence demonstrating that NTs enhance quantal neurotransmitter release at synapses through presynaptic mechanisms. The authors further propose that a potential target for NT actions at presynaptic terminals is the mechanism by which terminals retrieve synaptic vesicles after exocytosis. Depending on the temporal demands placed on synapses during high-frequency synaptic transmission, synapses may use two alternative modes of synaptic vesicle retrieval, the conventional slow endosomal recycling or a faster rapid retrieval at the active zone, referred to as "kiss-and-run." By modulating Ca2+ microdomains associated with voltage-gated Ca2+ channels at active zones, NTs may elicit a switch from the slow to the fast mode of endocytosis of vesicles at presynaptic terminals during high-frequency synaptic transmission, allowing more reliable information transfer and neuronal signaling in the central nervous system.
Figures
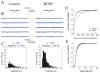
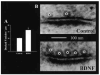
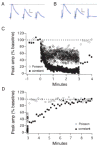
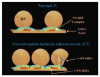
Similar articles
-
BDNF enhances quantal neurotransmitter release and increases the number of docked vesicles at the active zones of hippocampal excitatory synapses.J Neurosci. 2001 Jun 15;21(12):4249-58. doi: 10.1523/JNEUROSCI.21-12-04249.2001. J Neurosci. 2001. PMID: 11404410 Free PMC article.
-
The synaptic vesicle cycle.Annu Rev Neurosci. 2004;27:509-47. doi: 10.1146/annurev.neuro.26.041002.131412. Annu Rev Neurosci. 2004. PMID: 15217342 Review.
-
The synaptic vesicle cluster: a source of endocytic proteins during neurotransmitter release.Neuroscience. 2009 Jan 12;158(1):204-10. doi: 10.1016/j.neuroscience.2008.03.035. Epub 2008 Mar 26. Neuroscience. 2009. PMID: 18440714 Review.
-
Ca(2+) channels and transmitter release at the active zone.Cell Calcium. 2012 Sep-Oct;52(3-4):199-207. doi: 10.1016/j.ceca.2012.04.011. Epub 2012 Jun 8. Cell Calcium. 2012. PMID: 22682961 Review.
-
Mechanisms, locations, and kinetics of synaptic BDNF secretion: an update.Neurosci Res. 2009 Sep;65(1):11-22. doi: 10.1016/j.neures.2009.06.004. Epub 2009 Jun 11. Neurosci Res. 2009. PMID: 19523993
Cited by
-
Modulation of neuronal calcium signaling by neurotrophic factors.Int J Dev Neurosci. 2002 Jun-Aug;20(3-5):199-207. doi: 10.1016/s0736-5748(02)00014-x. Int J Dev Neurosci. 2002. PMID: 12175855 Free PMC article.
-
Hippocampal excitability increases during the estrous cycle in the rat: a potential role for brain-derived neurotrophic factor.J Neurosci. 2003 Dec 17;23(37):11641-52. doi: 10.1523/JNEUROSCI.23-37-11641.2003. J Neurosci. 2003. PMID: 14684866 Free PMC article.
-
Modulation of dendritic spine development and plasticity by BDNF and vesicular trafficking: fundamental roles in neurodevelopmental disorders associated with mental retardation and autism.J Neurodev Disord. 2009 Sep;1(3):185-96. doi: 10.1007/s11689-009-9027-6. J Neurodev Disord. 2009. PMID: 19966931 Free PMC article.
-
Homeostatic synaptic plasticity: from single synapses to neural circuits.Curr Opin Neurobiol. 2012 Jun;22(3):516-21. doi: 10.1016/j.conb.2011.09.006. Epub 2011 Oct 7. Curr Opin Neurobiol. 2012. PMID: 21983330 Free PMC article. Review.
-
BDNF increases release probability and the size of a rapidly recycling vesicle pool within rat hippocampal excitatory synapses.J Physiol. 2006 Aug 1;574(Pt 3):787-803. doi: 10.1113/jphysiol.2006.111310. Epub 2006 May 18. J Physiol. 2006. PMID: 16709633 Free PMC article.
References
-
- Abbott LF, Varela JA, Sen K, Nelson SB. Synaptic depression and cortical gain control. Science. 1997;275:220–4. - PubMed
-
- Abenavoli A, Montagna M, Malgaroli A. Calcium: the common theme in vesicular cycling. Nat Neurosci. 2001;4:117–8. - PubMed
-
- Augustine GJ. How does calcium trigger neurotransmitter release? Curr Opin Neurobiol. 2001;11:320–6. - PubMed
-
- Baldelli P, Forni PE, Carbone E. BDNF, NT-3 and NGF induce distinct new Ca2+ channel synthesis in developing hippocampal neurons. Eur J Neurosci. 2000;12:4017–32. - PubMed
-
- Baldelli P, Magnelli V, Carbone E. Selective up-regulation of P- and R-type Ca2+ channels in rat embryo motoneurons by BDNF. Eur J Neurosci. 1999;11:1127–33. - PubMed
Publication types
MeSH terms
Substances
Grants and funding
LinkOut - more resources
Full Text Sources
Research Materials
Miscellaneous