Localisation of the human hSuv3p helicase in the mitochondrial matrix and its preferential unwinding of dsDNA
- PMID: 12466530
- PMCID: PMC137961
- DOI: 10.1093/nar/gkf647
Localisation of the human hSuv3p helicase in the mitochondrial matrix and its preferential unwinding of dsDNA
Abstract
We characterised the human hSuv3p protein belonging to the family of NTPases/helicases. In yeast mitochondria the hSUV3 orthologue is a component of the degradosome complex and participates in mtRNA turnover and processing, while in Caenorhabditis elegans the hSUV3 orthologue is necessary for viability of early embryos. Using immunofluorescence analysis, an in vitro mitochondrial uptake assay and sub-fractionation of human mitochondria we show hSuv3p to be a soluble protein localised in the mitochondrial matrix. We expressed and purified recombinant hSuv3p protein from a bacterial expression system. The purified enzyme was capable of hydrolysing ATP with a K(m) of 41.9 micro M and the activity was only modestly stimulated by polynucleotides. hSuv3p unwound partly hybridised dsRNA and dsDNA structures with a very strong preference for the latter. The presented analysis of the hSuv3p NTPase/helicase suggests that new functions of the protein have been acquired in the course of evolution.
Figures
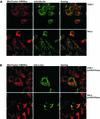
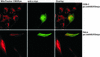
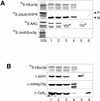
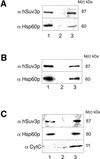
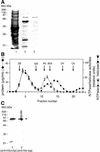
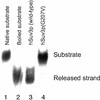
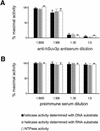
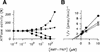
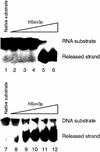
Similar articles
-
Human ATP-dependent RNA/DNA helicase hSuv3p interacts with the cofactor of survivin HBXIP.FEBS J. 2005 Oct;272(19):5008-19. doi: 10.1111/j.1742-4658.2005.04910.x. FEBS J. 2005. PMID: 16176273
-
Dbp9p, a member of the DEAD box protein family, exhibits DNA helicase activity.J Biol Chem. 2004 May 14;279(20):20692-8. doi: 10.1074/jbc.M400231200. Epub 2004 Mar 17. J Biol Chem. 2004. PMID: 15028736
-
Human mitochondrial RNA turnover caught in flagranti: involvement of hSuv3p helicase in RNA surveillance.Nucleic Acids Res. 2010 Jan;38(1):279-98. doi: 10.1093/nar/gkp903. Epub 2009 Oct 28. Nucleic Acids Res. 2010. PMID: 19864255 Free PMC article.
-
Yeast and human mitochondrial helicases.Biochim Biophys Acta. 2013 Aug;1829(8):842-53. doi: 10.1016/j.bbagrm.2013.02.009. Epub 2013 Feb 27. Biochim Biophys Acta. 2013. PMID: 23454114 Review.
-
RNA degradation in yeast and human mitochondria.Biochim Biophys Acta. 2012 Sep-Oct;1819(9-10):1027-34. doi: 10.1016/j.bbagrm.2011.11.010. Epub 2011 Dec 8. Biochim Biophys Acta. 2012. PMID: 22178375 Review.
Cited by
-
Human 2'-phosphodiesterase localizes to the mitochondrial matrix with a putative function in mitochondrial RNA turnover.Nucleic Acids Res. 2011 May;39(9):3754-70. doi: 10.1093/nar/gkq1282. Epub 2011 Jan 17. Nucleic Acids Res. 2011. PMID: 21245038 Free PMC article.
-
Nuclear-encoded factors involved in post-transcriptional processing and modification of mitochondrial tRNAs in human disease.Front Genet. 2015 Mar 10;6:79. doi: 10.3389/fgene.2015.00079. eCollection 2015. Front Genet. 2015. PMID: 25806043 Free PMC article. Review.
-
The process of mammalian mitochondrial protein synthesis.Cell Tissue Res. 2017 Jan;367(1):5-20. doi: 10.1007/s00441-016-2456-0. Epub 2016 Jul 14. Cell Tissue Res. 2017. PMID: 27411691 Free PMC article. Review.
-
Human mitochondrial degradosome prevents harmful mitochondrial R loops and mitochondrial genome instability.Proc Natl Acad Sci U S A. 2018 Oct 23;115(43):11024-11029. doi: 10.1073/pnas.1807258115. Epub 2018 Oct 9. Proc Natl Acad Sci U S A. 2018. PMID: 30301808 Free PMC article.
-
A Graphene-Based Platform for the Assay of Duplex-DNA Unwinding by Helicase.Angew Chem Weinheim Bergstr Ger. 2010 Aug 2;122(33):5839-5843. doi: 10.1002/ange.201001332. Epub 2010 Jul 7. Angew Chem Weinheim Bergstr Ger. 2010. PMID: 32313315 Free PMC article.
References
-
- Margossian S.P., Li,H., Zassenhaus,H.P. and Butow,R.A. (1996) The DexH box protein Suv3p is a component of a yeast mitochondrial 3′-to-5′ exoribonuclease that suppresses group I intron toxicity. Cell, 84, 199–209. - PubMed
-
- Dmochowska A., Golik,P. and Stepien,P.P. (1995) The novel nuclear gene DSS-1 of Saccharomyces cerevisiae is necessary for mitochondrial biogenesis. Curr. Genet., 28, 108–112. - PubMed
-
- Stepien P.P., Kokot,L., Leski,T. and Bartnik,E. (1995) The suv3 nuclear gene product is required for the in vivo processing of the yeast mitochondrial 21s rRNA transcripts containing the r1 intron. Curr. Genet., 27, 234–238. - PubMed
Publication types
MeSH terms
Substances
Grants and funding
LinkOut - more resources
Full Text Sources
Molecular Biology Databases