A novel function for human factor C1 (HCF-1), a host protein required for herpes simplex virus infection, in pre-mRNA splicing
- PMID: 12456665
- PMCID: PMC136956
- DOI: 10.1093/emboj/cdf652
A novel function for human factor C1 (HCF-1), a host protein required for herpes simplex virus infection, in pre-mRNA splicing
Abstract
Human factor C1 (HCF-1) is needed for the expression of herpes simplex virus 1 (HSV-1) immediate-early genes in infected mammalian cells. Here, we provide evidence that HCF-1 is required for spliceosome assembly and splicing in mammalian nuclear extracts. HCF-1 interacts with complexes containing splicing snRNPs in uninfected mammalian cells and is a stable component of the spliceosome complex. We show that a missense mutation in HCF-1 in the BHK21 hamster cell line tsBN67, at the non-permissive temperature, inhibits the protein's interaction with U1 and U5 splicing snRNPs, causes inefficient spliceosome assembly and inhibits splicing. Transient expression of wild-type HCF-1 in tsBN67 cells restores splicing at the non-permissive temperature. The inhibition of splicing in tsBN67 cells correlates with the temperature-sensitive cell cycle arrest phenotype, suggesting that HCF-1-dependent splicing events may be required for cell cycle progression.
Figures
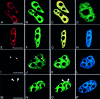
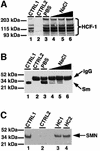
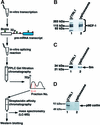
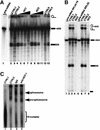
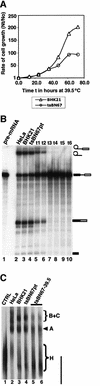
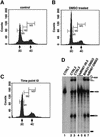
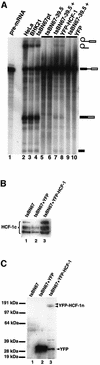
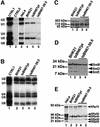
Similar articles
-
Spontaneous reversion of tsBN67 cell proliferation and cytokinesis defects in the absence of HCF-1 function.Exp Cell Res. 2002 Jul 1;277(1):119-30. doi: 10.1006/excr.2002.5551. Exp Cell Res. 2002. PMID: 12061822
-
Loss of HCF-1-chromatin association precedes temperature-induced growth arrest of tsBN67 cells.Mol Cell Biol. 2001 Jun;21(11):3820-9. doi: 10.1128/MCB.21.11.3820-3829.2001. Mol Cell Biol. 2001. PMID: 11340173 Free PMC article.
-
A single-point mutation in HCF causes temperature-sensitive cell-cycle arrest and disrupts VP16 function.Genes Dev. 1997 Mar 15;11(6):726-37. doi: 10.1101/gad.11.6.726. Genes Dev. 1997. PMID: 9087427
-
The spliceosome.Bioessays. 1993 Sep;15(9):595-603. doi: 10.1002/bies.950150905. Bioessays. 1993. PMID: 8240312 Review.
-
The dynamics of HCF-1 modulation of herpes simplex virus chromatin during initiation of infection.Viruses. 2013 May 22;5(5):1272-91. doi: 10.3390/v5051272. Viruses. 2013. PMID: 23698399 Free PMC article. Review.
Cited by
-
HCF-1 functions as a coactivator for the zinc finger protein Krox20.J Biol Chem. 2003 Dec 19;278(51):51116-24. doi: 10.1074/jbc.M303470200. Epub 2003 Oct 6. J Biol Chem. 2003. PMID: 14532282 Free PMC article.
-
Site-specific proteolysis of the transcriptional coactivator HCF-1 can regulate its interaction with protein cofactors.Proc Natl Acad Sci U S A. 2006 May 2;103(18):6817-22. doi: 10.1073/pnas.0602109103. Epub 2006 Apr 19. Proc Natl Acad Sci U S A. 2006. PMID: 16624878 Free PMC article.
-
FRET analyses of the U2AF complex localize the U2AF35/U2AF65 interaction in vivo and reveal a novel self-interaction of U2AF35.RNA. 2005 Aug;11(8):1201-14. doi: 10.1261/rna.7277705. RNA. 2005. PMID: 16043505 Free PMC article.
-
Systematic discovery of protein interaction interfaces using AlphaFold and experimental validation.Mol Syst Biol. 2024 Feb;20(2):75-97. doi: 10.1038/s44320-023-00005-6. Epub 2024 Jan 15. Mol Syst Biol. 2024. PMID: 38225382 Free PMC article.
-
Host cell factor-1 and E2F4 interact via multiple determinants in each protein.Mol Cell Biochem. 2006 Aug;288(1-2):79-90. doi: 10.1007/s11010-006-9122-x. Epub 2006 Apr 22. Mol Cell Biochem. 2006. PMID: 16633736
References
-
- Adams J., Kelso,R. and Cooley,L. (2000) The kelch superfamily of proteins: propellers of cell function. Trends Cell Biol., 10, 17–24. - PubMed
-
- Beales M., Flay,N., McKinney,R., Habara,Y., Ohshima,Y., Tani,T. and Potashkin,J. (2000) Mutations in the large subunit of U2AF disrupt pre-mRNA splicing, cell cycle progression and nuclear structure. Yeast, 16, 1001–1013. - PubMed
-
- Bentley D. (2002) The mRNA assembly line: transcription and processing machines in the same factory. Curr. Opin. Cell Biol., 14, 336–342. - PubMed
Publication types
MeSH terms
Substances
LinkOut - more resources
Full Text Sources
Medical