Kaposi's sarcoma-associated herpesvirus (human herpesvirus 8) replication and transcription factor activates the K9 (vIRF) gene through two distinct cis elements by a non-DNA-binding mechanism
- PMID: 12414946
- PMCID: PMC136869
- DOI: 10.1128/jvi.76.23.12044-12054.2002
Kaposi's sarcoma-associated herpesvirus (human herpesvirus 8) replication and transcription factor activates the K9 (vIRF) gene through two distinct cis elements by a non-DNA-binding mechanism
Abstract
The replication and transcription activator (RTA) of Kaposi's sarcoma-associated herpesvirus (KSHV), or human herpesvirus 8, a homologue of Epstein-Barr virus BRLF1 or Rta, is a strong transactivator and inducer of lytic replication. RTA acting alone can induce lytic replication of KSHV in infected cell lines that originated from primary effusion lymphomas, leading to virus production. During the lytic replication process, RTA activates many kinds of genes, including polyadenylated nuclear RNA, K8, K9 (vIRF), ORF57, and so on. We focused here on the mechanism of how RTA upregulates the K9 (vIRF) promoter and identified two independent cis-acting elements in the K9 (vIRF) promoter that responded to RTA. These elements were finally confined to the sequence 5'-TCTGGGACAGTC-3' in responsive element (RE) I-2B and the sequence 5'-GTACTTAAAATA-3' in RE IIC-2, both of which did not share sequence homology. Multiple factors bound specifically with these elements, and their binding was correlated with the RTA-responsive activity. Electrophoretic mobility shift assay with nuclear extract from infected cells and the N-terminal part of RTA expressed in Escherichia coli, however, did not show that RTA interacted directly with these elements, in contrast to the RTA responsive elements in the PAN/K12 promoter region, the ORF57/K8 promoter region. Thus, it was likely that RTA could transactivate several kinds of unique cis elements without directly binding to the responsive elements, probably through cooperation with other DNA-binding factors.
Figures
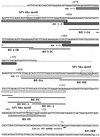
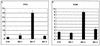
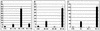
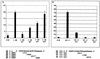
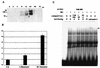
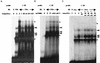
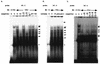
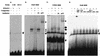
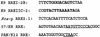
Similar articles
-
CCAAT/enhancer-binding protein-alpha is induced during the early stages of Kaposi's sarcoma-associated herpesvirus (KSHV) lytic cycle reactivation and together with the KSHV replication and transcription activator (RTA) cooperatively stimulates the viral RTA, MTA, and PAN promoters.J Virol. 2003 Sep;77(17):9590-612. doi: 10.1128/jvi.77.17.9590-9612.2003. J Virol. 2003. PMID: 12915572 Free PMC article.
-
Role of CCAAT/enhancer-binding protein alpha (C/EBPalpha) in activation of the Kaposi's sarcoma-associated herpesvirus (KSHV) lytic-cycle replication-associated protein (RAP) promoter in cooperation with the KSHV replication and transcription activator (RTA) and RAP.J Virol. 2003 Jan;77(1):600-23. doi: 10.1128/jvi.77.1.600-623.2003. J Virol. 2003. PMID: 12477864 Free PMC article.
-
Comparative study of regulation of RTA-responsive genes in Kaposi's sarcoma-associated herpesvirus/human herpesvirus 8.J Virol. 2003 Sep;77(17):9451-62. doi: 10.1128/jvi.77.17.9451-9462.2003. J Virol. 2003. PMID: 12915560 Free PMC article.
-
The Rta/Orf50 transactivator proteins of the gamma-herpesviridae.Curr Top Microbiol Immunol. 2007;312:71-100. doi: 10.1007/978-3-540-34344-8_3. Curr Top Microbiol Immunol. 2007. PMID: 17089794 Review.
-
Lytic cycle switches of oncogenic human gammaherpesviruses.Adv Cancer Res. 2007;97:81-109. doi: 10.1016/S0065-230X(06)97004-3. Adv Cancer Res. 2007. PMID: 17419942 Review.
Cited by
-
Binding of RBP-Jkappa (CSL) protein to the promoter of the Kaposi's sarcoma-associated herpesvirus ORF47 (gL) gene is a critical but not sufficient determinant of transactivation by ORF50 protein.Virology. 2010 Mar 1;398(1):38-48. doi: 10.1016/j.virol.2009.11.022. Epub 2009 Dec 16. Virology. 2010. PMID: 20006367 Free PMC article.
-
Kaposi's sarcoma-associated herpesvirus/human herpesvirus 8 ORF50/Rta lytic switch protein functions as a tetramer.J Virol. 2007 Jun;81(11):5788-806. doi: 10.1128/JVI.00140-07. Epub 2007 Mar 28. J Virol. 2007. PMID: 17392367 Free PMC article.
-
Upregulation of cellular Bcl-2 by the KSHV encoded RTA promotes virion production.PLoS One. 2011;6(8):e23892. doi: 10.1371/journal.pone.0023892. Epub 2011 Aug 25. PLoS One. 2011. PMID: 21901143 Free PMC article.
-
Molecular genetics of Kaposi's sarcoma-associated herpesvirus (human herpesvirus-8) epidemiology and pathogenesis.Microbiol Mol Biol Rev. 2003 Jun;67(2):175-212, table of contents. doi: 10.1128/MMBR.67.2.175-212.2003. Microbiol Mol Biol Rev. 2003. PMID: 12794189 Free PMC article. Review.
-
Kaposi's Sarcoma-associated herpesvirus lytic switch protein stimulates DNA binding of RBP-Jk/CSL to activate the Notch pathway.J Virol. 2006 Oct;80(19):9697-709. doi: 10.1128/JVI.00746-06. J Virol. 2006. PMID: 16973574 Free PMC article.
References
-
- Adamson, A. L., and S. C. Kenny. 1998. Rescue of the Epstein-Barr virus BZLF1 mutant, Z(S186A), early gene activation defect by the BRLF1 product. Virology 251:187-197. - PubMed
-
- Arvanitakis, L., E. A. Mesri, R. G. Nador, J. W. Said, A. S. Asch, D. M. Knowles, and E. Cesarman. 1996. Establishment and characterization of primary effusion (body cavity-based) lymphoma cell line (BC-3) harboring Kaposi's sarcoma-associated herpesvirus (KSHV/HHV8) in the absence of Epstein-Barr virus. Blood 88:2648-2654. - PubMed
-
- Cesarman, E., Y. Chang, P. S. Moore, J. W. Said, and D. M. Knowles. 1995. Kaposi's sarcoma-associated herpesvirus-like DNA sequences in AIDS-related body-cavity-based lymphomas. N. Engl. J. Med. 332:1186-1191. - PubMed
-
- Cesarman, E., P. S. Moore, P. H. Rao, G. Inghirami, D. M. Knowles, and Y. Chang. 1995. In vitro establishment and characterization of two acquired immunodeficiency syndrome-related lymphoma cell lines (BC-1 and BC-2) containing Kaposi's sarcoma-associated herpesvirus-like (KSHV) DNA sequences. Blood 86:2708-2714. - PubMed
-
- Chang, J., R. Rolf, D. Dittmer, and D. Ganem. 2000. Inflammatory cytokines and the reactivation of Kaposi's sarcoma-associated herpesvirus lytic replication. Virology 266:17-25. - PubMed
Publication types
MeSH terms
Substances
LinkOut - more resources
Full Text Sources
Research Materials