Rab32 is an A-kinase anchoring protein and participates in mitochondrial dynamics
- PMID: 12186851
- PMCID: PMC2174006
- DOI: 10.1083/jcb.200204081
Rab32 is an A-kinase anchoring protein and participates in mitochondrial dynamics
Abstract
A-kinase anchoring proteins (AKAPs) tether the cAMP-dependent protein kinase (PKA) and other signaling enzymes to distinct subcellular organelles. Using the yeast two-hybrid approach, we demonstrate that Rab32, a member of the Ras superfamily of small molecular weight G-proteins, interacts directly with the type II regulatory subunit of PKA. Cellular and biochemical studies confirm that Rab32 functions as an AKAP inside cells. Anchoring determinants for PKA have been mapped to sites within the conserved alpha5 helix that is common to all Rab family members. Subcellular fractionation and immunofluorescent approaches indicate that Rab32 and a proportion of the cellular PKA pool are associated with mitochondria. Transient transfection of a GTP binding-deficient mutant of Rab32 promotes aberrant accumulation of mitochondria at the microtubule organizing center. Further analysis of this mutant indicates that disruption of the microtubule cytoskeleton results in aberrantly elongated mitochondria. This implicates Rab32 as a participant in synchronization of mitochondrial fission. Thus, Rab32 is a dual function protein that participates in both mitochondrial anchoring of PKA and mitochondrial dynamics.
Figures
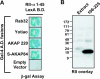
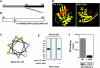
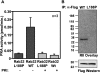
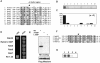
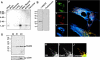
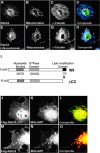
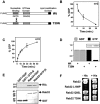
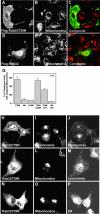
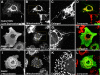
Similar articles
-
Rab32 regulates melanosome transport in Xenopus melanophores by protein kinase a recruitment.Curr Biol. 2007 Dec 4;17(23):2030-4. doi: 10.1016/j.cub.2007.10.051. Epub 2007 Nov 8. Curr Biol. 2007. PMID: 17997311 Free PMC article.
-
Analysis of A-kinase anchoring protein (AKAP) interaction with protein kinase A (PKA) regulatory subunits: PKA isoform specificity in AKAP binding.J Mol Biol. 2000 Apr 28;298(2):329-39. doi: 10.1006/jmbi.2000.3662. J Mol Biol. 2000. PMID: 10764601
-
An entirely specific type I A-kinase anchoring protein that can sequester two molecules of protein kinase A at mitochondria.Proc Natl Acad Sci U S A. 2011 Nov 29;108(48):E1227-35. doi: 10.1073/pnas.1107182108. Epub 2011 Nov 14. Proc Natl Acad Sci U S A. 2011. PMID: 22084075 Free PMC article.
-
The biological functions of A-kinase anchor proteins.J Mol Biol. 2001 Apr 27;308(2):99-114. doi: 10.1006/jmbi.2001.4585. J Mol Biol. 2001. PMID: 11327755 Review.
-
Mechanisms of protein kinase A anchoring.Int Rev Cell Mol Biol. 2010;283:235-330. doi: 10.1016/S1937-6448(10)83005-9. Int Rev Cell Mol Biol. 2010. PMID: 20801421 Review.
Cited by
-
Anchored PKA synchronizes adrenergic phosphoregulation of cardiac Cav1.2 channels.J Biol Chem. 2024 Sep;300(9):107656. doi: 10.1016/j.jbc.2024.107656. Epub 2024 Aug 10. J Biol Chem. 2024. PMID: 39128715 Free PMC article.
-
Strong selection signatures for Aleutian disease tolerance acting on novel candidate genes linked to immune and cellular responses in American mink (Neogale vison).Sci Rep. 2024 Jan 10;14(1):1035. doi: 10.1038/s41598-023-51039-7. Sci Rep. 2024. PMID: 38200094 Free PMC article.
-
Skeletal muscle proteome analysis underpins multifaceted mitochondrial dysfunction in Friedreich's ataxia.Front Neurosci. 2023 Oct 31;17:1289027. doi: 10.3389/fnins.2023.1289027. eCollection 2023. Front Neurosci. 2023. PMID: 38027498 Free PMC article.
-
Targeting small GTPases: emerging grasps on previously untamable targets, pioneered by KRAS.Signal Transduct Target Ther. 2023 May 23;8(1):212. doi: 10.1038/s41392-023-01441-4. Signal Transduct Target Ther. 2023. PMID: 37221195 Free PMC article. Review.
-
Rab32 promotes glioblastoma migration and invasion via regulation of ERK/Drp1-mediated mitochondrial fission.Cell Death Dis. 2023 Mar 15;14(3):198. doi: 10.1038/s41419-023-05721-3. Cell Death Dis. 2023. PMID: 36922509 Free PMC article.
References
-
- Bao, X., A.E. Faris, E.K. Jang, and R.J. Haslam. 2002. Molecular cloning, bacterial expression and properties of Rab31 and Rab32. Eur. J. Biochem. 269:259–271. - PubMed
-
- Bock, J.B., H.T. Matern, A.A. Peden, and R.H. Scheller. 2001. A genomic perspective on membrane compartment organization. Nature. 409:839–841. - PubMed
-
- Bourne, H.R., D.A. Sanders, and F. McCormick. 1991. The GTPase superfamily: conserved structure and molecular mechanism. Nature. 349:117–121. - PubMed
-
- Carr, D.W., and J.D. Scott. 1992. Blotting and band-shifting: techniques for studying protein-protein interactions. Trends Biochem. Sci. 17:246–249. - PubMed
-
- Carr, D.W., R.E. Stofko-Hahn, I.D.C. Fraser, S.M. Bishop, T.S. Acott, R.G. Brennan, and J.D. Scott. 1991. Interaction of the regulatory subunit (RII) of cAMP-dependent protein kinase with RII-anchoring proteins occurs through an amphipathic helix binding motif. J. Biol. Chem. 266:14188–14192. - PubMed
Publication types
MeSH terms
Substances
Grants and funding
LinkOut - more resources
Full Text Sources
Other Literature Sources
Molecular Biology Databases