Fast vesicle recycling supports neurotransmission during sustained stimulation at hippocampal synapses
- PMID: 11880491
- PMCID: PMC6758906
- DOI: 10.1523/JNEUROSCI.22-05-01608.2002
Fast vesicle recycling supports neurotransmission during sustained stimulation at hippocampal synapses
Abstract
High-frequency induced short-term synaptic depression is a common feature of central synapses in which synaptic responses rapidly decrease to a sustained level. A limitation in the availability of release-ready vesicles is thought to be a major factor underlying this phenomenon. Here, we studied the kinetics of vesicle reavailability and reuse during synaptic depression at hippocampal synapses. High-intensity stimulation of neurotransmitter release was induced by hyperosmolarity, high potassium, or action potential firing at 30 Hz to produce synaptic depression. Under these conditions, synaptic transmission rapidly depressed to a plateau level that was typically 10-40% of the initial response and persisted at this level for at least 5 min regardless of the developmental stage of synapses. This nondeclining phase of transmission was partly sustained by fast recycling and reuse of synaptic vesicles even after minutes of stimulation. Simultaneous electrical recording of postsynaptic responses and styryl dye destaining showed that after an initial round of exocytosis, vesicles were available for reuse with a delay between 1 and 3 sec during 30 Hz action potential or hypertonicity-induced stimulation. During these stimulation paradigms, there was a limited mobilization of vesicles from the reserve pool. During 10 Hz stimulation, however, the extent of vesicle reuse was minimal during the first 20 sec. These results suggest a role for fast vesicle recycling as a functional homeostatic mechanism that prevents vesicle depletion and maintains synaptic responses in the face of intense stimulation.
Figures
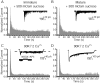
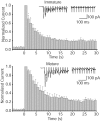
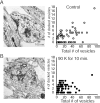
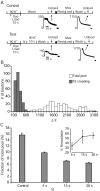
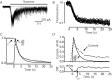
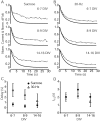
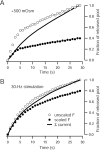
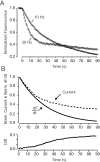
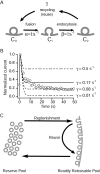
Similar articles
-
Rapid reuse of readily releasable pool vesicles at hippocampal synapses.Neuron. 2000 Oct;28(1):221-31. doi: 10.1016/s0896-6273(00)00098-2. Neuron. 2000. PMID: 11086996
-
VAMP4 Maintains a Ca2+-Sensitive Pool of Spontaneously Recycling Synaptic Vesicles.J Neurosci. 2020 Jul 8;40(28):5389-5401. doi: 10.1523/JNEUROSCI.2386-19.2020. Epub 2020 Jun 12. J Neurosci. 2020. PMID: 32532887 Free PMC article.
-
An isolated pool of vesicles recycles at rest and drives spontaneous neurotransmission.Neuron. 2005 Feb 17;45(4):563-73. doi: 10.1016/j.neuron.2004.12.056. Neuron. 2005. PMID: 15721242
-
Multiple vesicle recycling pathways in central synapses and their impact on neurotransmission.J Physiol. 2007 Dec 15;585(Pt 3):669-79. doi: 10.1113/jphysiol.2007.137745. Epub 2007 Aug 9. J Physiol. 2007. PMID: 17690145 Free PMC article. Review.
-
The synaptic vesicle cycle revisited.Neuron. 2000 Nov;28(2):317-20. doi: 10.1016/s0896-6273(00)00109-4. Neuron. 2000. PMID: 11144340 Review. No abstract available.
Cited by
-
Release Mode Dynamically Regulates the RRP Refilling Mechanism at Individual Hippocampal Synapses.J Neurosci. 2020 Oct 28;40(44):8426-8437. doi: 10.1523/JNEUROSCI.3029-19.2020. Epub 2020 Sep 28. J Neurosci. 2020. PMID: 32989096 Free PMC article.
-
Competition between phasic and asynchronous release for recovered synaptic vesicles at developing hippocampal autaptic synapses.J Neurosci. 2004 Jan 14;24(2):420-33. doi: 10.1523/JNEUROSCI.4452-03.2004. J Neurosci. 2004. PMID: 14724240 Free PMC article.
-
Borna disease virus blocks potentiation of presynaptic activity through inhibition of protein kinase C signaling.PLoS Pathog. 2006 Mar;2(3):e19. doi: 10.1371/journal.ppat.0020019. Epub 2006 Mar 17. PLoS Pathog. 2006. PMID: 16552443 Free PMC article.
-
Clathrin-mediated endocytosis at the synaptic terminal: bridging the gap between physiology and molecules.Traffic. 2010 Dec;11(12):1489-97. doi: 10.1111/j.1600-0854.2010.01104.x. Traffic. 2010. PMID: 20633242 Free PMC article. Review.
-
Protein kinase A-mediated synapsin I phosphorylation is a central modulator of Ca2+-dependent synaptic activity.J Neurosci. 2006 Nov 8;26(45):11670-81. doi: 10.1523/JNEUROSCI.3321-06.2006. J Neurosci. 2006. PMID: 17093089 Free PMC article.
References
-
- Bolshakov VY, Siegelbaum SA. Regulation of hippocampal transmitter release during development and long-term potentiation. Science. 1995;269:1730–1734. - PubMed
Publication types
MeSH terms
Substances
LinkOut - more resources
Full Text Sources
Other Literature Sources