Binding of tobramycin leads to conformational changes in yeast tRNA(Asp) and inhibition of aminoacylation
- PMID: 11847123
- PMCID: PMC125865
- DOI: 10.1093/emboj/21.4.760
Binding of tobramycin leads to conformational changes in yeast tRNA(Asp) and inhibition of aminoacylation
Abstract
Aminoglycosides inhibit translation in bacteria by binding to the A site in the ribosome. Here, it is shown that, in yeast, aminoglycosides can also interfere with other processes of translation in vitro. Steady-state aminoacylation kinetics of unmodified yeast tRNA(Asp) transcript indicate that the complex between tRNA(Asp) and tobramycin is a competitive inhibitor of the aspartylation reaction with an inhibition constant (K(I)) of 36 nM. Addition of an excess of heterologous tRNAs did not reverse the charging of tRNA(Asp), indicating a specific inhibition of the aspartylation reaction. Although magnesium ions compete with the inhibitory effect, the formation of the aspartate adenylate in the ATP-PP(i) exchange reaction by aspartyl-tRNA synthetase in the absence of the tRNA is not inhibited. Ultraviolet absorbance melting experiments indicate that tobramycin interacts with and destabilizes the native L-shaped tertiary structure of tRNA(Asp). Fluorescence anisotropy using fluorescein-labelled tobramycin reveals a stoichiometry of one molecule bound to tRNA(Asp) with a K(D) of 267 nM. The results indicate that aminoglycosides are biologically effective when their binding induces a shift in a conformational equilibrium of the RNA.
Figures
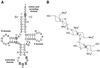
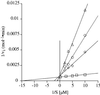
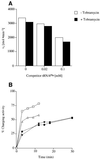
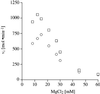
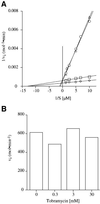
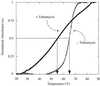
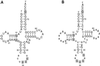
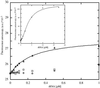
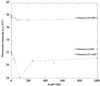
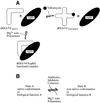
Similar articles
-
Yeast aspartyl-tRNA synthetase residues interacting with tRNA(Asp) identity bases connectively contribute to tRNA(Asp) binding in the ground and transition-state complex and discriminate against non-cognate tRNAs.J Mol Biol. 1999 Aug 27;291(4):761-73. doi: 10.1006/jmbi.1999.3012. J Mol Biol. 1999. PMID: 10452887
-
Complex ligand-induced conformational changes in tRNA(Asp) revealed by single-nucleotide resolution SHAPE chemistry.Biochemistry. 2008 Mar 18;47(11):3454-61. doi: 10.1021/bi702372x. Epub 2008 Feb 22. Biochemistry. 2008. PMID: 18290632
-
Efficient aminoacylation of a yeast tRNA(Asp) transcript with a 5' extension.FEBS Lett. 1990 Sep 17;270(1-2):4-8. doi: 10.1016/0014-5793(90)81221-9. FEBS Lett. 1990. PMID: 2226785
-
Exploring the aminoacylation function of transfer RNA by macromolecular engineering approaches. Involvement of conformational features in the charging process of yeast tRNA(Asp).Biochimie. 1990 Jun-Jul;72(6-7):453-61. doi: 10.1016/0300-9084(90)90069-s. Biochimie. 1990. PMID: 2124148 Review.
-
Aspartate identity of transfer RNAs.Biochimie. 1996;78(7):605-23. doi: 10.1016/s0300-9084(96)80007-1. Biochimie. 1996. PMID: 8955904 Review.
Cited by
-
tRNAs as antibiotic targets.Int J Mol Sci. 2014 Dec 25;16(1):321-49. doi: 10.3390/ijms16010321. Int J Mol Sci. 2014. PMID: 25547494 Free PMC article. Review.
-
An anti-tuberculosis compound screen using a zebrafish infection model identifies an aspartyl-tRNA synthetase inhibitor.Dis Model Mech. 2021 Dec 1;14(12):dmm049145. doi: 10.1242/dmm.049145. Epub 2021 Dec 23. Dis Model Mech. 2021. PMID: 34643222 Free PMC article.
-
Riboswitches: discovery of drugs that target bacterial gene-regulatory RNAs.Acc Chem Res. 2011 Dec 20;44(12):1329-38. doi: 10.1021/ar200039b. Epub 2011 May 26. Acc Chem Res. 2011. PMID: 21615107 Free PMC article. Review.
-
Pentamidine binds to tRNA through non-specific hydrophobic interactions and inhibits aminoacylation and translation.Nucleic Acids Res. 2008 Mar;36(5):1654-64. doi: 10.1093/nar/gkm1180. Epub 2008 Feb 7. Nucleic Acids Res. 2008. PMID: 18263620 Free PMC article.
-
Aminoacyl-tRNA Synthetases as Valuable Targets for Antimicrobial Drug Discovery.Int J Mol Sci. 2021 Feb 10;22(4):1750. doi: 10.3390/ijms22041750. Int J Mol Sci. 2021. PMID: 33578647 Free PMC article. Review.
References
-
- Blanchard S.C., Fourmy,D., Eason,R.G. and Puglisi,J.D. (1998) rRNA chemical groups required for aminoglycoside binding. Biochemistry, 37, 7716–7724. - PubMed
-
- Cavarelli J., Rees,B., Ruff,M., Thierry,J.C. and Moras,D. (1993) Yeast tRNA(Asp) recognition by its cognate class II aminoacyl-tRNA synthetase. Nature, 362, 181–184. - PubMed
-
- Frugier M., Söll,D., Giegé,R. and Florentz,C. (1994) Identity switches between tRNAs aminoacylated by class I glutaminyl- and class II aspartyl-tRNA synthetases. Biochemistry, 33, 9912–9921. - PubMed
-
- Gangloff J., Keith,G., Ebel,J.P. and Dirheimer,G. (1971) Structure of aspartate-tRNA from brewer’s yeast. Nature New Biol., 230, 125–126. - PubMed
Publication types
MeSH terms
Substances
LinkOut - more resources
Full Text Sources
Other Literature Sources
Molecular Biology Databases
Miscellaneous