Endoplasmic reticulum-Golgi intermediate compartment membranes and vimentin filaments participate in vaccinia virus assembly
- PMID: 11799179
- PMCID: PMC135913
- DOI: 10.1128/jvi.76.4.1839-1855.2002
Endoplasmic reticulum-Golgi intermediate compartment membranes and vimentin filaments participate in vaccinia virus assembly
Abstract
Vaccinia virus (VV) has a complex morphogenetic pathway whose first steps are poorly characterized. We have studied the early phase of VV assembly, when viral factories and spherical immature viruses (IVs) form in the cytoplasm of the infected cell. After freeze-substitution numerous cellular elements are detected around assembling viruses: membranes, ribosomes, microtubules, filaments, and unidentified structures. A double membrane is clearly resolved in the VV envelope for the first time, and freeze fracture reveals groups of tubules interacting laterally on the surface of the viroplasm foci. These data strongly support the hypothesis of a cellular tubulovesicular compartment, related to the endoplasmic reticulum-Golgi intermediate compartment (ERGIC), as the origin of the first VV envelope. Moreover, the cytoskeletal vimentin intermediate filaments are found around viral factories and inside the viroplasm foci, where vimentin and the VV core protein p39 colocalize in the areas where crescents protrude. Confocal microscopy showed that ERGIC elements and vimentin filaments concentrate in the viral factories. We propose that modified cellular ERGIC membranes and vimentin intermediate filaments act coordinately in the construction of viral factories and the first VV form through a unique mechanism of viral morphogenesis from cellular elements.
Figures
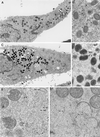
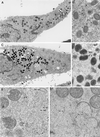
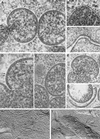
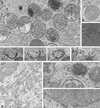
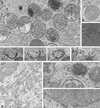
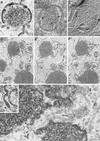
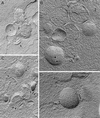
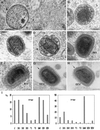
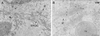
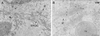
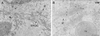
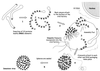
Similar articles
-
Direct formation of vaccinia virus membranes from the endoplasmic reticulum in the absence of the newly characterized L2-interacting protein A30.5.J Virol. 2013 Nov;87(22):12313-26. doi: 10.1128/JVI.02137-13. Epub 2013 Sep 11. J Virol. 2013. PMID: 24027302 Free PMC article.
-
Vaccinia virus 15-kilodalton (A14L) protein is essential for assembly and attachment of viral crescents to virosomes.J Virol. 1998 Feb;72(2):1287-96. doi: 10.1128/JVI.72.2.1287-1296.1998. J Virol. 1998. PMID: 9445029 Free PMC article.
-
Evidence against an essential role of COPII-mediated cargo transport to the endoplasmic reticulum-Golgi intermediate compartment in the formation of the primary membrane of vaccinia virus.J Virol. 2003 Nov;77(21):11754-66. doi: 10.1128/jvi.77.21.11754-11766.2003. J Virol. 2003. PMID: 14557660 Free PMC article.
-
Poxvirus membrane biogenesis.Virology. 2015 May;479-480:619-26. doi: 10.1016/j.virol.2015.02.003. Epub 2015 Feb 26. Virology. 2015. PMID: 25728299 Free PMC article. Review.
-
Assembly of vaccinia virus revisited: de novo membrane synthesis or acquisition from the host?Trends Microbiol. 2002 Jan;10(1):15-24. doi: 10.1016/s0966-842x(01)02256-9. Trends Microbiol. 2002. PMID: 11755081 Review.
Cited by
-
Direct formation of vaccinia virus membranes from the endoplasmic reticulum in the absence of the newly characterized L2-interacting protein A30.5.J Virol. 2013 Nov;87(22):12313-26. doi: 10.1128/JVI.02137-13. Epub 2013 Sep 11. J Virol. 2013. PMID: 24027302 Free PMC article.
-
Poxvirus membrane biogenesis: rupture not disruption.Cell Microbiol. 2013 Feb;15(2):190-9. doi: 10.1111/cmi.12072. Epub 2012 Dec 16. Cell Microbiol. 2013. PMID: 23168015 Free PMC article. Review.
-
Mapping and functional analysis of interaction sites within the cytoplasmic domains of the vaccinia virus A33R and A36R envelope proteins.J Virol. 2003 Apr;77(7):4113-26. doi: 10.1128/jvi.77.7.4113-4126.2003. J Virol. 2003. PMID: 12634370 Free PMC article.
-
Vaccinia virus entry into cells is dependent on a virion surface protein encoded by the A28L gene.J Virol. 2004 Mar;78(5):2357-66. doi: 10.1128/jvi.78.5.2357-2366.2004. J Virol. 2004. PMID: 14963132 Free PMC article.
-
The E6 protein from vaccinia virus is required for the formation of immature virions.Virology. 2010 Apr 10;399(2):201-11. doi: 10.1016/j.virol.2010.01.012. Epub 2010 Feb 8. Virology. 2010. PMID: 20116821 Free PMC article.
References
-
- Appenzeller, C., H. Andersson, F. Kappeler, and H.-P. Hauri. 1999. The lectin ERGIC-53 is a cargo transport receptor for glycoproteins. Nat. Cell Biol. 1:330-334. - PubMed
-
- Baumeister, W., and A. C. Steven. 2000. Macromolecular electron microscopy in the era of structural genomics. Trends Biochem. Sci. 25:624-631. - PubMed
Publication types
MeSH terms
Substances
LinkOut - more resources
Full Text Sources
Other Literature Sources