Intracellular trafficking by Star regulates cleavage of the Drosophila EGF receptor ligand Spitz
- PMID: 11799065
- PMCID: PMC155325
- DOI: 10.1101/gad.214202
Intracellular trafficking by Star regulates cleavage of the Drosophila EGF receptor ligand Spitz
Abstract
Spitz (Spi) is a TGFalpha homolog that is a cardinal ligand for the Drosophila EGF receptor throughout development. Cleavage of the ubiquitously expressed transmembrane form of Spi (mSpi) precedes EGF receptor activation. We show that the Star and Rhomboid (Rho) proteins are necessary for Spi cleavage in Drosophila cells. Complexes between the Spi and Star proteins, as well as between the Star and Rho proteins were identified, but no Spi-Star-Rho triple complex was detected. This observation suggests a sequential activity of Star and Rho in mSpi processing. The interactions between Spi and Star regulate the intracellular trafficking of Spi. The Spi precursor is retained in the periphery of the nucleus. Coexpression of Star promotes translocation of Spi to a compartment where Rho is present both in cells and in embryos. A Star deletion construct that maintains binding to Spi and Rho, but is unable to facilitate Spi translocation, lost biological activity. These results underscore the importance of regulated intracellular trafficking in processing of a TGFalpha family ligand.
Figures
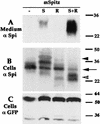
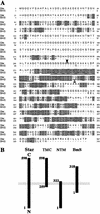
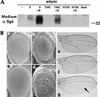
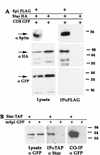
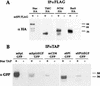
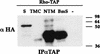
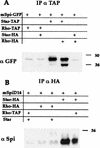
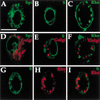
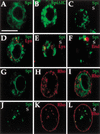
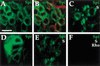
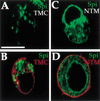
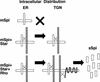
Similar articles
-
Rhomboid and Star facilitate presentation and processing of the Drosophila TGF-alpha homolog Spitz.Genes Dev. 2000 Jan 15;14(2):177-86. Genes Dev. 2000. PMID: 10652272 Free PMC article.
-
Keren, a new ligand of the Drosophila epidermal growth factor receptor, undergoes two modes of cleavage.EMBO J. 2002 Aug 15;21(16):4287-96. doi: 10.1093/emboj/cdf439. EMBO J. 2002. PMID: 12169631 Free PMC article.
-
Rhomboid cleaves Star to regulate the levels of secreted Spitz.EMBO J. 2007 Mar 7;26(5):1211-20. doi: 10.1038/sj.emboj.7601581. Epub 2007 Feb 15. EMBO J. 2007. PMID: 17304216 Free PMC article.
-
EGF receptor signalling: the importance of presentation.Curr Biol. 2000 May 18;10(10):R388-91. doi: 10.1016/s0960-9822(00)00485-1. Curr Biol. 2000. PMID: 10837218 Review.
-
Developmental roles of Rhomboid proteases.Semin Cell Dev Biol. 2016 Dec;60:5-9. doi: 10.1016/j.semcdb.2016.07.014. Epub 2016 Jul 14. Semin Cell Dev Biol. 2016. PMID: 27423914 Review.
Cited by
-
How Drosophila melanogaster Forms its Mechanoreceptors.Curr Genomics. 2008;9(5):312-23. doi: 10.2174/138920208785133271. Curr Genomics. 2008. PMID: 19471605 Free PMC article.
-
An Entamoeba histolytica rhomboid protease with atypical specificity cleaves a surface lectin involved in phagocytosis and immune evasion.Genes Dev. 2008 Jun 15;22(12):1636-46. doi: 10.1101/gad.1667708. Genes Dev. 2008. PMID: 18559479 Free PMC article.
-
EGFR/ARF6 regulation of Hh signalling stimulates oncogenic Ras tumour overgrowth.Nat Commun. 2017 Mar 10;8:14688. doi: 10.1038/ncomms14688. Nat Commun. 2017. PMID: 28281543 Free PMC article.
-
The Drosophila jing gene is a downstream target in the Trachealess/Tango tracheal pathway.Dev Genes Evol. 2010 Dec;220(7-8):191-206. doi: 10.1007/s00427-010-0339-z. Dev Genes Evol. 2010. PMID: 21061019
-
RNAi screen of endoplasmic reticulum-associated host factors reveals a role for IRE1alpha in supporting Brucella replication.PLoS Pathog. 2008 Jul 25;4(7):e1000110. doi: 10.1371/journal.ppat.1000110. PLoS Pathog. 2008. PMID: 18654626 Free PMC article.
References
-
- Bier E, Jan LY, Jan YN. rhomboid, a gene required for dorsoventral axis establishment and peripheral nervous system development in Drosophila melanogaster. Genes & Dev. 1990;4:190–203. - PubMed
-
- Eggenschwiler JT, Espinoza E, Anderson KV. Rab23 is an essential negative regulator of the mouse Sonic hedgehog signalling pathway. Nature. 2001;412:194–198. - PubMed
-
- Ellgaard L, Molinari M, Helenius A. Setting the standards: Quality control in the secretory pathway. Science. 1999;286:1882–1888. - PubMed
Publication types
MeSH terms
Substances
LinkOut - more resources
Full Text Sources
Other Literature Sources
Molecular Biology Databases
Miscellaneous