Plasma membrane proton ATPase Pma1p requires raft association for surface delivery in yeast
- PMID: 11739806
- PMCID: PMC60781
- DOI: 10.1091/mbc.12.12.4129
Plasma membrane proton ATPase Pma1p requires raft association for surface delivery in yeast
Abstract
Correct sorting of proteins is essential to generate and maintain the identity and function of the different cellular compartments. In this study we demonstrate the role of lipid rafts in biosynthetic delivery of Pma1p, the major plasma membrane proton ATPase, to the cell surface. Disruption of rafts led to mistargeting of Pma1p to the vacuole. Conversely, Pma1-7, an ATPase mutant that is mistargeted to the vacuole, was shown to exhibit impaired raft association. One of the previously identified suppressors, multicopy AST1, not only restored surface delivery but also raft association of Pma1-7. Ast1p, which is a peripheral membrane protein, was found to directly interact with Pma1p inducing its clustering into a SDS/Triton X100-resistant oligomer. We suggest that clustering facilitates partition of Pma1p into rafts and transport to the cell surface.
Figures
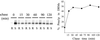
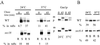
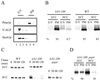
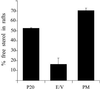
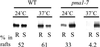
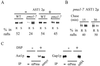
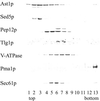
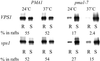
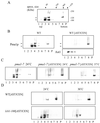
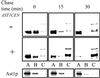
Similar articles
-
Lactoferrin perturbs lipid rafts and requires integrity of Pma1p-lipid rafts association to exert its antifungal activity against Saccharomyces cerevisiae.Int J Biol Macromol. 2021 Feb 28;171:343-357. doi: 10.1016/j.ijbiomac.2020.12.224. Epub 2021 Jan 7. Int J Biol Macromol. 2021. PMID: 33421469
-
Ubiquitin-mediated targeting of a mutant plasma membrane ATPase, Pma1-7, to the endosomal/vacuolar system in yeast.Mol Biol Cell. 2004 May;15(5):2401-9. doi: 10.1091/mbc.e03-10-0727. Epub 2004 Mar 12. Mol Biol Cell. 2004. PMID: 15020711 Free PMC article.
-
Very long-chain fatty acid-containing lipids rather than sphingolipids per se are required for raft association and stable surface transport of newly synthesized plasma membrane ATPase in yeast.J Biol Chem. 2006 Nov 10;281(45):34135-45. doi: 10.1074/jbc.M603791200. Epub 2006 Sep 15. J Biol Chem. 2006. PMID: 16980694
-
Lipid-dependent surface transport of the proton pumping ATPase: a model to study plasma membrane biogenesis in yeast.Biochimie. 2007 Feb;89(2):249-54. doi: 10.1016/j.biochi.2006.07.020. Epub 2006 Aug 15. Biochimie. 2007. PMID: 16938383 Review.
-
The yeast Pma1 proton pump: a model for understanding the biogenesis of plasma membrane proteins.J Biol Chem. 2001 Aug 10;276(32):29613-6. doi: 10.1074/jbc.R100022200. Epub 2001 Jun 12. J Biol Chem. 2001. PMID: 11404364 Review. No abstract available.
Cited by
-
The joint action of yeast eisosomes and membraneless organelles in response to ethanol stress.Heliyon. 2024 May 19;10(10):e31561. doi: 10.1016/j.heliyon.2024.e31561. eCollection 2024 May 30. Heliyon. 2024. PMID: 38818138 Free PMC article.
-
Shared and more specific genetic determinants and pathways underlying yeast tolerance to acetic, butyric, and octanoic acids.Microb Cell Fact. 2024 Feb 29;23(1):71. doi: 10.1186/s12934-024-02309-0. Microb Cell Fact. 2024. PMID: 38419072 Free PMC article.
-
Ergosterol distribution controls surface structure formation and fungal pathogenicity.mBio. 2023 Aug 31;14(4):e0135323. doi: 10.1128/mbio.01353-23. Epub 2023 Jul 6. mBio. 2023. PMID: 37409809 Free PMC article.
-
Ergosterol distribution controls surface structure formation and fungal pathogenicity.bioRxiv [Preprint]. 2023 Feb 17:2023.02.17.528979. doi: 10.1101/2023.02.17.528979. bioRxiv. 2023. Update in: mBio. 2023 Aug 31;14(4):e0135323. doi: 10.1128/mbio.01353-23. PMID: 36824733 Free PMC article. Updated. Preprint.
-
Giardial lipid rafts share virulence factors with secreted vesicles and participate in parasitic infection in mice.Front Cell Infect Microbiol. 2022 Aug 23;12:974200. doi: 10.3389/fcimb.2022.974200. eCollection 2022. Front Cell Infect Microbiol. 2022. PMID: 36081774 Free PMC article.
References
-
- Ambesi A, Miranda M, Petrov VV, Slayman CW. Biogenesis and function of the yeast plasma-membrane H(+)-ATPase. J Exp Biol. 2000;203(Pt 1):155–160. - PubMed
-
- Benito B, Moreno E, Lagunas R. Half-life of the plasma membrane ATPase and its activating system in resting yeast cells. Biochim Biophys Acta. 1991;1063:265–268. - PubMed
-
- Brown DA, Rose JK. Sorting of GPI-anchored proteins to glycolipid-enriched membrane subdomains during transport to the apical cell surface. Cell. 1992;68:533–544. - PubMed
Publication types
MeSH terms
Substances
Grants and funding
LinkOut - more resources
Full Text Sources
Molecular Biology Databases