Tyrosine phosphorylation of protein kinase Cdelta is essential for its apoptotic effect in response to etoposide
- PMID: 11739733
- PMCID: PMC134204
- DOI: 10.1128/MCB.22.1.182-195.2002
Tyrosine phosphorylation of protein kinase Cdelta is essential for its apoptotic effect in response to etoposide
Abstract
Protein kinase Cdelta (PKCdelta) is involved in the apoptosis of various cells in response to diverse stimuli. In this study, we characterized the role of PKCdelta in the apoptosis of C6 glioma cells in response to etoposide. We found that etoposide induced apoptosis in the C6 cells within 24 to 48 h and arrested the cells in the G(1)/S phase of the cell cycle. Overexpression of PKCdelta increased the apoptotic effect induced by etoposide, whereas the PKCdelta selective inhibitor rottlerin and the PKCdelta dominant-negative mutant K376R reduced this effect compared to control cells. Etoposide-induced tyrosine phosphorylation of PKCdelta and its translocation to the nucleus within 3 h was followed by caspase-dependent cleavage of the enzyme. Using PKC chimeras, we found that both the regulatory and catalytic domains of PKCdelta were necessary for its apoptotic effect. The role of tyrosine phosphorylation of PKCdelta in the effects of etoposide was examined using cells overexpressing a PKCdelta mutant in which five tyrosine residues were mutated to phenylalanine (PKCdelta5). These cells exhibited decreased apoptosis in response to etoposide compared to cells overexpressing PKCdelta. Likewise, activation of caspase 3 and the cleavage of the PKCdelta5 mutant were significantly lower in cells overexpressing PKCdelta5. Using mutants of PKCdelta altered at individual tyrosine residues, we identified tyrosine 64 and tyrosine 187 as important phosphorylation sites in the apoptotic effect induced by etoposide. Our results suggest a role of PKCdelta in the apoptosis induced by etoposide and implicate tyrosine phosphorylation of PKCdelta as an important regulator of this effect.
Figures
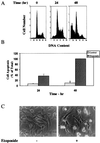
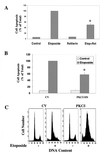
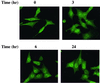
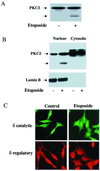
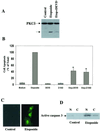
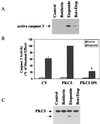
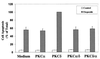
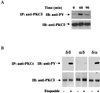
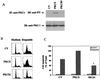
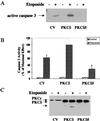
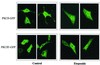
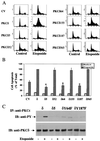
Similar articles
-
Caspase-3-dependent proteolytic cleavage of protein kinase Cdelta is essential for oxidative stress-mediated dopaminergic cell death after exposure to methylcyclopentadienyl manganese tricarbonyl.J Neurosci. 2002 Mar 1;22(5):1738-51. doi: 10.1523/JNEUROSCI.22-05-01738.2002. J Neurosci. 2002. PMID: 11880503 Free PMC article.
-
Protein kinase C delta is essential for etoposide-induced apoptosis in salivary gland acinar cells.J Biol Chem. 1999 Jul 2;274(27):19115-23. doi: 10.1074/jbc.274.27.19115. J Biol Chem. 1999. PMID: 10383415
-
Protein kinase Cdelta amplifies ceramide formation via mitochondrial signaling in prostate cancer cells.J Clin Invest. 2002 Mar;109(6):827-36. doi: 10.1172/JCI14146. J Clin Invest. 2002. PMID: 11901191 Free PMC article.
-
Protein kinase Cdelta and apoptosis.Biochem Soc Trans. 2007 Nov;35(Pt 5):1001-4. doi: 10.1042/BST0351001. Biochem Soc Trans. 2007. PMID: 17956263 Review.
-
Role of proteolytic activation of protein kinase Cdelta in oxidative stress-induced apoptosis.Antioxid Redox Signal. 2003 Oct;5(5):609-20. doi: 10.1089/152308603770310275. Antioxid Redox Signal. 2003. PMID: 14580317 Review.
Cited by
-
Multifunctional roles of PKCδ: Opportunities for targeted therapy in human disease.Pharmacol Ther. 2016 Sep;165:1-13. doi: 10.1016/j.pharmthera.2016.05.001. Epub 2016 May 11. Pharmacol Ther. 2016. PMID: 27179744 Free PMC article. Review.
-
Involvement of protein kinase C-delta in DNA damage-induced apoptosis.J Cell Mol Med. 2003 Oct-Dec;7(4):341-50. doi: 10.1111/j.1582-4934.2003.tb00237.x. J Cell Mol Med. 2003. PMID: 14754503 Free PMC article. Review.
-
Protein kinase Cδ and caspase-3 modulate TRAIL-induced apoptosis in breast tumor cells.J Cell Biochem. 2010 Nov 1;111(4):979-87. doi: 10.1002/jcb.22786. J Cell Biochem. 2010. PMID: 20665667 Free PMC article.
-
Protein kinase C delta-mediated processes in cholecystokinin-8-stimulated pancreatic acini.Pancreas. 2009 Nov;38(8):930-5. doi: 10.1097/MPA.0b013e3181b8476a. Pancreas. 2009. PMID: 19752773 Free PMC article.
-
Etoposide induces protein kinase Cdelta- and caspase-3-dependent apoptosis in neuroblastoma cancer cells.Mol Pharmacol. 2009 Sep;76(3):632-40. doi: 10.1124/mol.109.054999. Epub 2009 Jun 23. Mol Pharmacol. 2009. PMID: 19549763 Free PMC article.
References
-
- Acs, P., K. Bogi, P. S. Lorenzo, A. M. Marquez, T. Biro, Z. Szallasi, and P. M. Blumberg. 1997. The catalytic domain of protein kinase C chimeras modulates the affinity and targeting of phorbol ester-induced translocation. J. Biol. Chem. 272:22148–22153. - PubMed
-
- Acs, P., Q. J. Wang, K. Bogi, A. M. Marquez, P. S. Lorenzo, T. Biro, Z. Szallasi, J. F. Mushinski, and P. M. Blumberg. 1997. Both the catalytic and regulatory domains of protein kinase C chimeras modulate the proliferative properties of NIH 3T3 cells. J. Biol. Chem. 272:28793–28799. - PubMed
-
- Bharti, A., S. K. Kraeft, M. Gounder, P. Pandey, S. Jin, Z. M. Yuan, S. P. Lees-Miller, R. Weichselbaum, D. Weaver, L. B. Chen, D. Kufe, and S. Kharbanda. 1998. Inactivation of DNA-dependent protein kinase by protein kinase Cδ: implications for apoptosis. Mol. Cell. Biol. 18:6719–6728. - PMC - PubMed
-
- Blake, R. A., P. Garcia-Paramio, P. J. Parker, and S. A. Courtneidge. 1999. Src promotes PKCδ degradation. Cell Growth Differ. 10:231–241. - PubMed
-
- Brodie, C., K. Bogi, P. Acs, P. S. Lorenzo, L. Baskin, and P. M. Blumberg. 1998. Protein kinase Cδ (PKCδ) inhibits the expression of glutamine synthetase in glial cells via the PKCδ regulatory domain and its tyrosine phosphorylation. J. Biol. Chem. 273:30713–30718. - PubMed
Publication types
MeSH terms
Substances
LinkOut - more resources
Full Text Sources
Other Literature Sources
Molecular Biology Databases
Research Materials