Vaccinia virus intracellular movement is associated with microtubules and independent of actin tails
- PMID: 11689647
- PMCID: PMC114752
- DOI: 10.1128/JVI.75.23.11651-11663.2001
Vaccinia virus intracellular movement is associated with microtubules and independent of actin tails
Abstract
Two mechanisms have been proposed for the intracellular movement of enveloped vaccinia virus virions: rapid actin polymerization and microtubule association. The first mechanism is used by the intracellular pathogens Listeria and Shigella, and the second is used by cellular vesicles transiting from the Golgi network to the plasma membrane. To distinguish between these models, two recombinant vaccinia viruses that express the B5R membrane protein fused to enhanced green fluorescent protein (GFP) were constructed. One had Tyr(112) and Tyr(132) of the A36R membrane protein, which are required for phosphorylation and the nucleation of actin tails, conservatively changed to Phe residues; the other had the A36R open reading frame deleted. Although the Tyr mutant was impaired in Tyr phosphorylation and actin tail formation, digital video and time-lapse confocal microscopy demonstrated that virion movement from the juxtanuclear region to the periphery was saltatory with maximal speeds of >2 microm/s and was inhibited by the microtubule-depolymerizing drug nocodazole. Moreover, this actin tail-independent movement was indistinguishable from that of a control virus with an unmutated A36R gene and closely resembled the movement of vesicles on microtubules. However, in the absence of actin tails, the Tyr mutant did not induce the formation of motile, virus-tipped microvilli and had a reduced ability to spread from cell to cell. The deletion mutant was more severely impaired, suggesting that the A36R protein has additional roles. Optical sections of unpermeabilized, B5R antibody-stained cells that expressed GFP-actin and were infected with wild-type vaccinia virus revealed that all actin tails were associated with virions on the cell surface. We concluded that the intracellular movement of intracellular enveloped virions occurs on microtubules and that the motile actin tails enhance extracellular virus spread to neighboring cells.
Figures
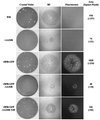
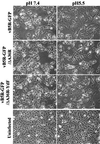
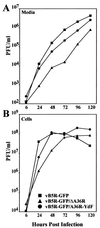
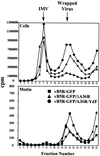
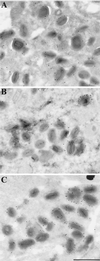
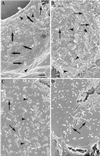
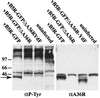
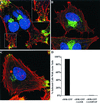
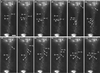
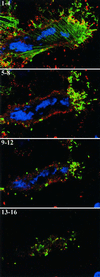
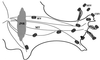
Similar articles
-
Visualization of intracellular movement of vaccinia virus virions containing a green fluorescent protein-B5R membrane protein chimera.J Virol. 2001 May;75(10):4802-13. doi: 10.1128/JVI.75.10.4802-4813.2001. J Virol. 2001. PMID: 11312352 Free PMC article.
-
Mutations in the vaccinia virus A33R and B5R envelope proteins that enhance release of extracellular virions and eliminate formation of actin-containing microvilli without preventing tyrosine phosphorylation of the A36R protein.J Virol. 2003 Nov;77(22):12266-75. doi: 10.1128/jvi.77.22.12266-12275.2003. J Virol. 2003. PMID: 14581563 Free PMC article.
-
Movements of vaccinia virus intracellular enveloped virions with GFP tagged to the F13L envelope protein.J Gen Virol. 2001 Nov;82(Pt 11):2747-2760. doi: 10.1099/0022-1317-82-11-2747. J Gen Virol. 2001. PMID: 11602786
-
The exit of vaccinia virus from infected cells.Virus Res. 2004 Dec;106(2):189-97. doi: 10.1016/j.virusres.2004.08.015. Virus Res. 2004. PMID: 15567497 Review.
-
Pox, dyes, and videotape: making movies of GFP-labeled vaccinia virus.Methods Mol Biol. 2004;269:205-18. doi: 10.1385/1-59259-789-0:205. Methods Mol Biol. 2004. PMID: 15114018 Review.
Cited by
-
Molecular genetic analysis of orf virus: a poxvirus that has adapted to skin.Viruses. 2015 Mar 23;7(3):1505-39. doi: 10.3390/v7031505. Viruses. 2015. PMID: 25807056 Free PMC article. Review.
-
Similarities in the induction of post-Golgi vesicles by the vaccinia virus F13L protein and phospholipase D.J Virol. 2002 Aug;76(15):7777-89. doi: 10.1128/jvi.76.15.7777-7789.2002. J Virol. 2002. PMID: 12097590 Free PMC article.
-
Induction of cell-cell fusion by ectromelia virus is not inhibited by its fusion inhibitory complex.Virol J. 2009 Sep 29;6:151. doi: 10.1186/1743-422X-6-151. Virol J. 2009. PMID: 19785778 Free PMC article.
-
Rho'ing in and out of cells: viral interactions with Rho GTPase signaling.Small GTPases. 2014;5:e28318. doi: 10.4161/sgtp.28318. Epub 2014 Mar 24. Small GTPases. 2014. PMID: 24691164 Free PMC article. Review.
-
Analysis of viral membranes formed in cells infected by a vaccinia virus L2-deletion mutant suggests their origin from the endoplasmic reticulum.J Virol. 2013 Feb;87(3):1861-71. doi: 10.1128/JVI.02779-12. Epub 2012 Nov 28. J Virol. 2013. PMID: 23192873 Free PMC article.
References
MeSH terms
Substances
LinkOut - more resources
Full Text Sources
Other Literature Sources