Interactions between NADPH oxidase-related proton and electron currents in human eosinophils
- PMID: 11559774
- PMCID: PMC2278831
- DOI: 10.1111/j.1469-7793.2001.00767.x
Interactions between NADPH oxidase-related proton and electron currents in human eosinophils
Abstract
1. Proton and electron currents in human eosinophils were studied using the permeabilized-patch voltage-clamp technique, with an applied NH4+ gradient to control pH(i). 2. Voltage-gated proton channels in unstimulated human eosinophils studied with the permeabilized-patch approach had properties similar to those reported in whole-cell studies. 3. Superoxide anion (O2-) release assessed by cytochrome c reduction was compared in human eosinophils and neutrophils stimulated by phorbol myristate acetate (PMA). PMA-stimulated O2 release was more transient and the maximum rate was three times greater in eosinophils. 4. In PMA-activated eosinophils, the H+ current amplitude (I(H)) at +60 mV increased 4.7-fold, activation was 4.0 times faster, deactivation (tail current decay) was 5.4 times slower, the H+ conductance-voltage (g(H)-V) relationship was shifted -43 mV, and diphenylene iodinium (DPI)-inhibitable inward current reflecting electron flow through NADPH oxidase was activated. The data reveal that PMA activates the H+ efflux during the respiratory burst by modulating the properties of H+ channels, not simply as a result of NADPH oxidase activity. 5. The electrophysiological response of eosinophils to PMA resembled that reported in human neutrophils, but PMA activated larger proton and electron currents in eosinophils and the response was more transient. 6. ZnCl2 slowed the activation of H+ currents and shifted the g(H)-V relationship to more positive voltages. These effects occurred at similar ZnCl2 concentrations in eosinophils before and after PMA stimulation. These data are compatible with the existence of a single type of H+ channel in eosinophils that is modulated during the respiratory burst.
Figures
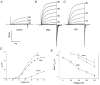
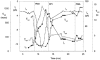
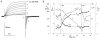
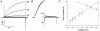
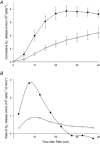
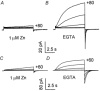
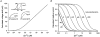
Similar articles
-
Activation of NADPH oxidase-related proton and electron currents in human eosinophils by arachidonic acid.J Physiol. 2001 Sep 15;535(Pt 3):783-94. doi: 10.1111/j.1469-7793.2001.00783.x. J Physiol. 2001. PMID: 11559775 Free PMC article.
-
Simultaneous activation of NADPH oxidase-related proton and electron currents in human neutrophils.Proc Natl Acad Sci U S A. 2000 Jun 6;97(12):6885-9. doi: 10.1073/pnas.100047297. Proc Natl Acad Sci U S A. 2000. PMID: 10823889 Free PMC article.
-
Interactions between electron and proton currents in excised patches from human eosinophils.J Gen Physiol. 2003 Dec;122(6):713-26. doi: 10.1085/jgp.200308891. J Gen Physiol. 2003. PMID: 14638931 Free PMC article.
-
Voltage-gated proton channels in microglia.Prog Neurobiol. 2001 Jun;64(3):277-305. doi: 10.1016/s0301-0082(00)00062-9. Prog Neurobiol. 2001. PMID: 11240310 Review.
-
Pharmacology of voltage-gated proton channels.Curr Pharm Des. 2007;13(23):2400-20. doi: 10.2174/138161207781368675. Curr Pharm Des. 2007. PMID: 17692009 Review.
Cited by
-
Voltage-gated proton channels.Compr Physiol. 2012 Apr;2(2):1355-85. doi: 10.1002/cphy.c100071. Compr Physiol. 2012. PMID: 23798303 Free PMC article. Review.
-
Voltage-gated proton channels: molecular biology, physiology, and pathophysiology of the H(V) family.Physiol Rev. 2013 Apr;93(2):599-652. doi: 10.1152/physrev.00011.2012. Physiol Rev. 2013. PMID: 23589829 Free PMC article. Review.
-
Biophysical properties of the voltage gated proton channel H(V)1.Wiley Interdiscip Rev Membr Transp Signal. 2012 Sep 1;1(5):605-620. doi: 10.1002/wmts.55. Epub 2012 May 11. Wiley Interdiscip Rev Membr Transp Signal. 2012. PMID: 23050239 Free PMC article.
-
Philosophy of voltage-gated proton channels.J R Soc Interface. 2013 Dec 18;11(92):20130799. doi: 10.1098/rsif.2013.0799. Print 2014 Mar 6. J R Soc Interface. 2013. PMID: 24352668 Free PMC article. Review.
-
Identification of a vacuolar proton channel that triggers the bioluminescent flash in dinoflagellates.PLoS One. 2017 Feb 8;12(2):e0171594. doi: 10.1371/journal.pone.0171594. eCollection 2017. PLoS One. 2017. PMID: 28178296 Free PMC article.
References
-
- Babior BM. NADPH oxidase: an update. Blood. 1999;93:1464–1476. - PubMed
-
- Cherny VV, DeCoursey AG, Henderson LM, Thomas LL, DeCoursey TE. Proton and electron currents during the respiratory burst in human eosinophils. Biophysical Journal. 2001a;80:506–507a. (abstract)
Publication types
MeSH terms
Substances
Grants and funding
LinkOut - more resources
Full Text Sources