Force and kinetic barriers to unzipping of the DNA double helix
- PMID: 11447279
- PMCID: PMC37483
- DOI: 10.1073/pnas.151257598
Force and kinetic barriers to unzipping of the DNA double helix
Abstract
A theory of the unzipping of double-stranded DNA is presented and is compared to recent micromanipulation experiments. It is shown that the interactions that stabilize the double helix and the elastic rigidity of single strands simply determine the sequence-dependent approximately 12-pN force threshold for DNA strand separation. Using a semimicroscopic model of the binding between nucleotide strands, we show that the greater rigidity of the strands when formed into double-stranded DNA, relative to that of isolated strands, gives rise to a potential barrier to unzipping. The effects of this barrier are derived analytically. The force to keep the extremities of the molecule at a fixed distance, the kinetic rates for strand unpairing at fixed applied force, and the rupture force as a function of loading rate are calculated. The dependence of the kinetics and of the rupture force on molecule length is also analyzed.
Figures
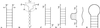
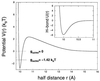
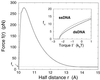
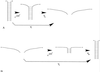
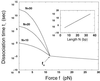
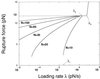
Similar articles
-
Force and kinetic barriers to initiation of DNA unzipping.Phys Rev E Stat Nonlin Soft Matter Phys. 2002 Apr;65(4 Pt 1):041907. doi: 10.1103/PhysRevE.65.041907. Epub 2002 Mar 28. Phys Rev E Stat Nonlin Soft Matter Phys. 2002. PMID: 12005873
-
Unzipping dynamics of long DNAs.Phys Rev E Stat Nonlin Soft Matter Phys. 2002 Nov;66(5 Pt 1):051914. doi: 10.1103/PhysRevE.66.051914. Epub 2002 Nov 22. Phys Rev E Stat Nonlin Soft Matter Phys. 2002. PMID: 12513530
-
Can a double stranded DNA be unzipped by pulling a single strand?: phases of adsorbed DNA.J Chem Phys. 2009 Apr 14;130(14):145105. doi: 10.1063/1.3116082. J Chem Phys. 2009. PMID: 19368478
-
Force-induced desorption and unzipping of semiflexible polymers.Phys Rev Lett. 2006 Aug 4;97(5):058302. doi: 10.1103/PhysRevLett.97.058302. Epub 2006 Aug 4. Phys Rev Lett. 2006. PMID: 17026145 Review.
-
Nucleic Acid Thermodynamics Derived from Mechanical Unzipping Experiments.Life (Basel). 2022 Jul 20;12(7):1089. doi: 10.3390/life12071089. Life (Basel). 2022. PMID: 35888177 Free PMC article. Review.
Cited by
-
DNA Nanotechnology for Investigating Mechanical Signaling in the Immune System.Angew Chem Int Ed Engl. 2023 Jul 24;62(30):e202302967. doi: 10.1002/anie.202302967. Epub 2023 May 15. Angew Chem Int Ed Engl. 2023. PMID: 37186502 Free PMC article. Review.
-
Energy coupling mechanisms of AcrB-like RND transporters.Biophys Rep. 2017;3(4):73-84. doi: 10.1007/s41048-017-0042-y. Epub 2017 Sep 25. Biophys Rep. 2017. PMID: 29238744 Free PMC article.
-
Force as a useful variable in reactions: unfolding RNA.Annu Rev Biophys Biomol Struct. 2004;33:363-85. doi: 10.1146/annurev.biophys.33.110502.140418. Annu Rev Biophys Biomol Struct. 2004. PMID: 15139818 Free PMC article. Review.
-
Pause point spectra in DNA constant-force unzipping.Biophys J. 2005 Apr;88(4):2752-65. doi: 10.1529/biophysj.104.047340. Epub 2005 Feb 4. Biophys J. 2005. PMID: 15695634 Free PMC article.
-
Tracing entire operation cycles of molecular motor hepatitis C virus helicase in structurally resolved dynamical simulations.Proc Natl Acad Sci U S A. 2010 Dec 7;107(49):20875-80. doi: 10.1073/pnas.1014631107. Epub 2010 Nov 16. Proc Natl Acad Sci U S A. 2010. PMID: 21081697 Free PMC article.
References
Publication types
MeSH terms
Substances
LinkOut - more resources
Full Text Sources