Human immunodeficiency virus type 1 (HIV-1)-induced GRO-alpha production stimulates HIV-1 replication in macrophages and T lymphocytes
- PMID: 11390582
- PMCID: PMC114296
- DOI: 10.1128/JVI.75.13.5812-5822.2001
Human immunodeficiency virus type 1 (HIV-1)-induced GRO-alpha production stimulates HIV-1 replication in macrophages and T lymphocytes
Abstract
We examined the early effects of infection by CCR5-using (R5 human immunodeficiency virus [HIV]) and CXCR4-using (X4 HIV) strains of HIV type 1 (HIV-1) on chemokine production by primary human monocyte-derived macrophages (MDM). While R5 HIV, but not X4 HIV, replicated in MDM, we found that the production of the C-X-C chemokine growth-regulated oncogene alpha (GRO-alpha) was markedly stimulated by X4 HIV and, to a much lesser extent, by R5 HIV. HIV-1 gp120 engagement of CXCR4 initiated the stimulation of GRO-alpha production, an effect blocked by antibodies to CXCR4. GRO-alpha then fed back and stimulated HIV-1 replication in both MDM and lymphocytes, and antibodies that neutralize GRO-alpha or CXCR2 (the receptor for GRO-alpha) markedly reduced viral replication in MDM and peripheral blood mononuclear cells. Therefore, activation of MDM by HIV-1 gp120 engagement of CXCR4 initiates an autocrine-paracrine loop that may be important in disease progression after the emergence of X4 HIV.
Figures
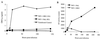
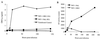
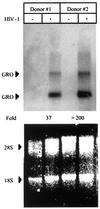
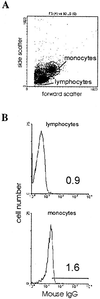
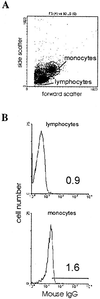
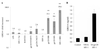
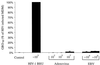
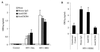
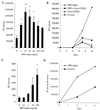
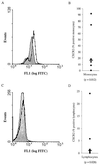
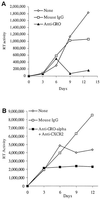
Similar articles
-
Interleukin-8 stimulates human immunodeficiency virus type 1 replication and is a potential new target for antiretroviral therapy.J Virol. 2001 Sep;75(17):8195-202. doi: 10.1128/jvi.75.17.8195-8202.2001. J Virol. 2001. PMID: 11483765 Free PMC article.
-
Complex determinants in human immunodeficiency virus type 1 envelope gp120 mediate CXCR4-dependent infection of macrophages.J Virol. 2005 Nov;79(21):13250-61. doi: 10.1128/JVI.79.21.13250-13261.2005. J Virol. 2005. PMID: 16227248 Free PMC article.
-
Redirecting migration of T cells to chemokine secreted from tumors by genetic modification with CXCR2.Hum Gene Ther. 2002 Nov 1;13(16):1971-80. doi: 10.1089/10430340260355374. Hum Gene Ther. 2002. PMID: 12427307
-
The macrophage response to HIV-1: Intracellular control of X4 virus replication accompanied by activation of chemokine and cytokine synthesis.J Neurovirol. 2002 Dec;8(6):599-610. doi: 10.1080/13550280290100923. J Neurovirol. 2002. PMID: 12476353 Review.
-
Macrophage activation through CCR5- and CXCR4-mediated gp120-elicited signaling pathways.J Leukoc Biol. 2003 Nov;74(5):676-82. doi: 10.1189/jlb.0503206. Epub 2003 Jul 22. J Leukoc Biol. 2003. PMID: 12960231 Review.
Cited by
-
Interleukin-8 stimulates human immunodeficiency virus type 1 replication and is a potential new target for antiretroviral therapy.J Virol. 2001 Sep;75(17):8195-202. doi: 10.1128/jvi.75.17.8195-8202.2001. J Virol. 2001. PMID: 11483765 Free PMC article.
-
Tissue-like environments shape functional interactions of HIV-1 with immature dendritic cells.EMBO Rep. 2023 Jun 5;24(6):e56818. doi: 10.15252/embr.202356818. Epub 2023 Apr 12. EMBO Rep. 2023. PMID: 37042686 Free PMC article.
-
High Abundance of genus Prevotella in the gut of perinatally HIV-infected children is associated with IP-10 levels despite therapy.Sci Rep. 2018 Dec 5;8(1):17679. doi: 10.1038/s41598-018-35877-4. Sci Rep. 2018. PMID: 30518941 Free PMC article.
-
Interleukin-8 and growth-regulated oncogene alpha mediate angiogenesis in Kaposi's sarcoma.J Virol. 2002 Nov;76(22):11570-83. doi: 10.1128/jvi.76.22.11570-11583.2002. J Virol. 2002. PMID: 12388718 Free PMC article.
-
ELR+ CXC chemokine expression in benign and malignant colorectal conditions.BMC Cancer. 2008 Jun 25;8:178. doi: 10.1186/1471-2407-8-178. BMC Cancer. 2008. PMID: 18578857 Free PMC article.
References
-
- Ahuja S K, Murphy P M. The CXC chemokines growth-regulated oncogene (GRO)α, GROβ, GROγ, neutrophil-activating peptide-2, and epithelial cell-derived neutrophil-activating peptide-78 are potent agonists for the type B, but not the type A, human interleukin-8 receptor. J Biol Chem. 1996;271:20545–20550. - PubMed
-
- Alkhatib G, Combadiere C, Broder C C, Feng Y, Kennedy P E, Murphy P M, Berger E A. CC CKR5: a RANTES, MIP-1α, MIP-1β receptor as a fusion cofactor for macrophage-tropic HIV-1. Science. 1996;272:1955–1958. - PubMed
-
- Amara A, Gall S L, Schwartz O, Salamero J, Montes M, Loetscher P, Baggiolini M, Virelizier J L, Arenzana-Seisdedos F. HIV coreceptor downregulation as antiviral principle: SDF-1α-dependent internalization of the chemokine receptor CXCR4 contributes to inhibition of HIV replication. J Exp Med. 1997;186:139–146. - PMC - PubMed
Publication types
MeSH terms
Substances
Grants and funding
LinkOut - more resources
Full Text Sources
Other Literature Sources