Regulation of glutamate dehydrogenase by reversible ADP-ribosylation in mitochondria
- PMID: 11350929
- PMCID: PMC125451
- DOI: 10.1093/emboj/20.10.2404
Regulation of glutamate dehydrogenase by reversible ADP-ribosylation in mitochondria
Abstract
Mitochondrial ADP-ribosylation leads to modification of two proteins of approximately 26 and 53 kDA: The nature of these proteins and, hence, the physiological consequences of their modification have remained unknown. Here, a 55 kDa protein, glutamate dehydrogenase (GDH), was established as a specific acceptor for enzymatic, cysteine-specific ADP-ribosylation in mitochondria. The modified protein was isolated from the mitochondrial preparation and identified as GDH by N-terminal sequencing and mass spectrometric analyses of tryptic digests. Incubation of human hepatoma cells with [14C]adenine demonstrated the occurrence of the modification in vivo. Purified GDH was ADP-ribosylated in a cysteine residue in the presence of the mitochondrial activity that transferred the ADP-ribose from NAD+ onto the acceptor site. ADP- ribosylation of GDH led to substantial inhibition of its catalytic activity. The stoichiometry between incorporated ADP-ribose and GDH subunits suggests that modification of one subunit per catalytically active homohexamer causes the inactivation of the enzyme. Isolated, ADP-ribosylated GDH was reactivated by an Mg2+-dependent mitochondrial ADP-ribosylcysteine hydrolase. GDH, a highly regulated enzyme, is the first mitochondrial protein identified whose activity may be modulated by ADP-ribosylation.
Figures
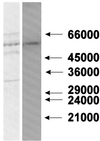
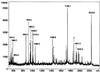
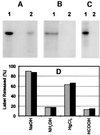
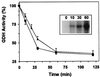
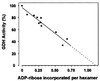
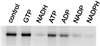
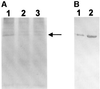
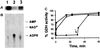
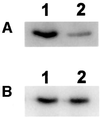
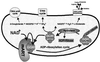
Similar articles
-
Identification of ADP-ribosylation site in human glutamate dehydrogenase isozymes.FEBS Lett. 2005 Aug 1;579(19):4125-30. doi: 10.1016/j.febslet.2005.06.041. FEBS Lett. 2005. PMID: 16023112
-
Enzymic, cysteine-specific ADP-ribosylation in bovine liver mitochondria.Biochem J. 1998 May 15;332 ( Pt 1)(Pt 1):189-93. doi: 10.1042/bj3320189. Biochem J. 1998. PMID: 9576867 Free PMC article.
-
Structural studies on ADP activation of mammalian glutamate dehydrogenase and the evolution of regulation.Biochemistry. 2003 Apr 1;42(12):3446-56. doi: 10.1021/bi0206917. Biochemistry. 2003. PMID: 12653548
-
NAD and ADP-ribose metabolism in mitochondria.FEBS J. 2013 Aug;280(15):3530-41. doi: 10.1111/febs.12304. Epub 2013 Jun 3. FEBS J. 2013. PMID: 23617329 Review.
-
Phospho adenylylation and phospho ADP-ribosylation, types of covalent protein modification derived from NADP.Biofactors. 1988 Jan;1(1):37-40. Biofactors. 1988. PMID: 3151246 Review.
Cited by
-
Isoform-specific targeting and interaction domains in human nicotinamide mononucleotide adenylyltransferases.J Biol Chem. 2010 Jun 11;285(24):18868-76. doi: 10.1074/jbc.M110.107631. Epub 2010 Apr 13. J Biol Chem. 2010. PMID: 20388704 Free PMC article.
-
MonoADP-ribosylation of the NAD+-dependent alcohol dehydrogenase from Entamoeba histolytica.Curr Microbiol. 2005 Sep;51(3):171-4. doi: 10.1007/s00284-005-4538-1. Epub 2005 Aug 2. Curr Microbiol. 2005. PMID: 16086106
-
Role of Nicotinamide Adenine Dinucleotide and Related Precursors as Therapeutic Targets for Age-Related Degenerative Diseases: Rationale, Biochemistry, Pharmacokinetics, and Outcomes.Antioxid Redox Signal. 2019 Jan 10;30(2):251-294. doi: 10.1089/ars.2017.7269. Epub 2018 May 11. Antioxid Redox Signal. 2019. PMID: 29634344 Free PMC article. Review.
-
The power to reduce: pyridine nucleotides--small molecules with a multitude of functions.Biochem J. 2007 Mar 1;402(2):205-18. doi: 10.1042/BJ20061638. Biochem J. 2007. PMID: 17295611 Free PMC article. Review.
-
SIRT4 and Its Roles in Energy and Redox Metabolism in Health, Disease and During Exercise.Front Physiol. 2019 Aug 9;10:1006. doi: 10.3389/fphys.2019.01006. eCollection 2019. Front Physiol. 2019. PMID: 31447696 Free PMC article. Review.
References
-
- Aktories K. (1997) Rho proteins: targets for bacterial toxins. Trends Microbiol., 5, 282–288. - PubMed
-
- Boyer P.D. (1997) The ATP synthase—a splendid molecular machine. Annu. Rev. Biochem., 66, 717–749. - PubMed
-
- Cervantes-Laurean D., Minter,D.E., Jacobson,E.L. and Jacobson,M.K. (1993) Protein glycation by ADP-ribose: studies of model conjugates. Biochemistry, 32, 1528–1534. - PubMed
-
- Cervantes-Laurean D., Loflin,P.T., Minter,D.E., Jacobson,E.L. and Jacobson,M.K. (1995) Protein modification by ADP-ribose via acid-labile linkages. J. Biol. Chem., 270, 7929–7936. - PubMed
-
- Chaurand P., Luetzenkirchen,F. and Spengler,B. (1999) Peptide and protein identification by matrix-assisted laser desorption ionization (MALDI) and MALDI-post-source decay time-of-flight mass spectrometry. J. Am. Soc. Mass Spectrom., 10, 91–103. - PubMed
Publication types
MeSH terms
Substances
LinkOut - more resources
Full Text Sources