Nuclear factor TDP-43 and SR proteins promote in vitro and in vivo CFTR exon 9 skipping
- PMID: 11285240
- PMCID: PMC145463
- DOI: 10.1093/emboj/20.7.1774
Nuclear factor TDP-43 and SR proteins promote in vitro and in vivo CFTR exon 9 skipping
Abstract
Alternative splicing of human cystic fibrosis transmembrane conductance regulator (CFTR) exon 9 is regulated by a combination of cis-acting elements distributed through the exon and both flanking introns (IVS8 and IVS9). Several studies have identified in the IVS8 intron 3' splice site a regulatory element that is composed of a polymorphic (TG)m(T)n repeated sequence. At present, no cellular factors have been identified that recognize this element. We have identified TDP-43, a nuclear protein not previously described to bind RNA, as the factor binding specifically to the (TG)m sequence. Transient TDP-43 overexpression in Hep3B cells results in an increase in exon 9 skipping. This effect is more pronounced with concomitant overexpression of SR proteins. Antisense inhibition of endogenous TDP-43 expression results in increased inclusion of exon 9, providing a new therapeutic target to correct aberrant splicing of exon 9 in CF patients. The clinical and biological relevance of this finding in vivo is demonstrated by our characterization of a CF patient carrying a TG10T9(DeltaF508)/TG13T3(wt) genotype leading to a disease-causing high proportion of exon 9 skipping.
Figures
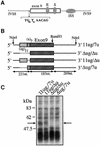
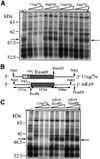
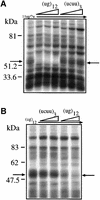
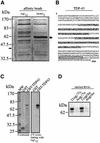
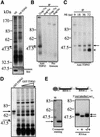
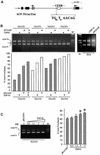
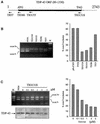
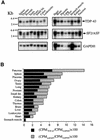
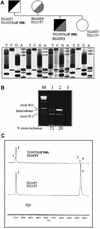
Similar articles
-
An intronic polypyrimidine-rich element downstream of the donor site modulates cystic fibrosis transmembrane conductance regulator exon 9 alternative splicing.J Biol Chem. 2004 Apr 23;279(17):16980-8. doi: 10.1074/jbc.M313439200. Epub 2004 Feb 13. J Biol Chem. 2004. PMID: 14966131
-
Characterization and functional implications of the RNA binding properties of nuclear factor TDP-43, a novel splicing regulator of CFTR exon 9.J Biol Chem. 2001 Sep 28;276(39):36337-43. doi: 10.1074/jbc.M104236200. Epub 2001 Jul 24. J Biol Chem. 2001. PMID: 11470789
-
Splicing factors induce cystic fibrosis transmembrane regulator exon 9 skipping through a nonevolutionary conserved intronic element.J Biol Chem. 2000 Jul 14;275(28):21041-7. doi: 10.1074/jbc.M910165199. J Biol Chem. 2000. PMID: 10766763
-
SR protein-mediated inhibition of CFTR exon 9 inclusion: molecular characterization of the intronic splicing silencer.Nucleic Acids Res. 2007;35(13):4359-68. doi: 10.1093/nar/gkm444. Epub 2007 Jun 18. Nucleic Acids Res. 2007. PMID: 17576688 Free PMC article.
-
CELF proteins regulate CFTR pre-mRNA splicing: essential role of the divergent domain of ETR-3.Nucleic Acids Res. 2010 Nov;38(20):7273-85. doi: 10.1093/nar/gkq573. Epub 2010 Jul 14. Nucleic Acids Res. 2010. PMID: 20631008 Free PMC article.
Cited by
-
Two mutations G335D and Q343R within the amyloidogenic core region of TDP-43 influence its aggregation and inclusion formation.Sci Rep. 2016 Mar 31;6:23928. doi: 10.1038/srep23928. Sci Rep. 2016. PMID: 27030292 Free PMC article.
-
Cellular model of TAR DNA-binding protein 43 (TDP-43) aggregation based on its C-terminal Gln/Asn-rich region.J Biol Chem. 2012 Mar 2;287(10):7512-25. doi: 10.1074/jbc.M111.288720. Epub 2012 Jan 10. J Biol Chem. 2012. PMID: 22235134 Free PMC article.
-
TDP-43 regulates global translational yield by splicing of exon junction complex component SKAR.Nucleic Acids Res. 2012 Mar;40(6):2668-82. doi: 10.1093/nar/gkr1082. Epub 2011 Nov 25. Nucleic Acids Res. 2012. PMID: 22121224 Free PMC article.
-
Mutant TDP-43 in motor neurons promotes the onset and progression of ALS in rats.J Clin Invest. 2012 Jan;122(1):107-18. doi: 10.1172/JCI59130. Epub 2011 Dec 12. J Clin Invest. 2012. PMID: 22156203 Free PMC article.
-
Tau mis-splicing in the pathogenesis of neurodegenerative disorders.BMB Rep. 2016 Aug;49(8):405-13. doi: 10.5483/bmbrep.2016.49.8.084. BMB Rep. 2016. PMID: 27222125 Free PMC article. Review.
References
-
- Chillon M. et al. (1995) Mutations in the cystic fibrosis gene in patients with congenital absence of the vas deferens. N. Engl. J. Med., 332, 1475–1480. - PubMed
-
- Chu C.S., Trapnell,B.C., Curristin,S., Cutting,G.R. and Crystal,R.G. (1993) Genetic basis of variable exon 9 skipping in cystic fibrosis transmembrane conductance regulator mRNA. Nature Genet., 3, 151–156. - PubMed
-
- Cockrill B.A. and Hales,C.A. (1999) Allergic bronchopulmonary aspergillosis. Annu. Rev. Med., 50, 303–316. - PubMed
-
- Cohn J.A., Friedman,K.J., Noone,P.G., Knowles,M.R., Silverman,L.M. and Jowell,P.S. (1998) Relation between mutations of the cystic fibrosis gene and idiopathic pancreatitis. N. Engl. J. Med., 339, 653–658. - PubMed
Publication types
MeSH terms
Substances
Grants and funding
LinkOut - more resources
Full Text Sources
Other Literature Sources
Molecular Biology Databases
Research Materials
Miscellaneous