Kinetic analysis of translocation through nuclear pore complexes
- PMID: 11250898
- PMCID: PMC145537
- DOI: 10.1093/emboj/20.6.1320
Kinetic analysis of translocation through nuclear pore complexes
Abstract
The mechanism of facilitated translocation through nuclear pore complexes (NPCs) is only poorly understood. Here, we present a kinetic analysis of the process using various model substrates. We find that the translocation capacity of NPCs is unexpectedly high, with a single NPC allowing a mass flow of nearly 100 MDa/s and rates in the order of 10(3) translocation events per second. Our data further indicate that high affinity interactions between the translocation substrate and NPC components are dispensable for translocation. We propose a 'selective phase model' that could explain how NPCs function as a permeability barrier for inert molecules and yet become selectively permeable for nuclear transport receptors and receptor-cargo complexes.
Figures
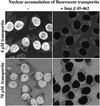
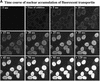
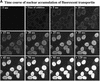
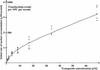
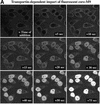
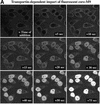
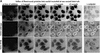
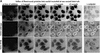
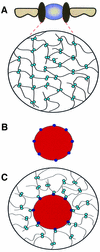
Similar articles
-
Nuclear transport of single molecules: dwell times at the nuclear pore complex.J Cell Biol. 2005 Jan 17;168(2):233-43. doi: 10.1083/jcb.200411005. J Cell Biol. 2005. PMID: 15657394 Free PMC article.
-
Nuclear protein import is reduced in cells expressing nuclear envelopathy-causing lamin A mutants.Exp Cell Res. 2009 Aug 15;315(14):2373-85. doi: 10.1016/j.yexcr.2009.05.003. Epub 2009 May 12. Exp Cell Res. 2009. PMID: 19442658
-
Characterization of Ran-driven cargo transport and the RanGTPase system by kinetic measurements and computer simulation.EMBO J. 2003 Mar 3;22(5):1088-100. doi: 10.1093/emboj/cdg113. EMBO J. 2003. PMID: 12606574 Free PMC article.
-
Dynamic nuclear pore complexes: life on the edge.Cell. 2006 Jun 16;125(6):1041-53. doi: 10.1016/j.cell.2006.05.027. Cell. 2006. PMID: 16777596 Review.
-
Should I stay or should I go? Nucleocytoplasmic trafficking in plant innate immunity.Cell Microbiol. 2007 Aug;9(8):1880-90. doi: 10.1111/j.1462-5822.2007.00962.x. Epub 2007 May 15. Cell Microbiol. 2007. PMID: 17506817 Review.
Cited by
-
A physical model describing the interaction of nuclear transport receptors with FG nucleoporin domain assemblies.Elife. 2016 Apr 8;5:e14119. doi: 10.7554/eLife.14119. Elife. 2016. PMID: 27058170 Free PMC article.
-
An introduction to dynamic nucleoporins in Leishmania species: Novel targets for tropical-therapeutics.J Parasit Dis. 2022 Dec;46(4):1176-1191. doi: 10.1007/s12639-022-01515-0. Epub 2022 Jul 25. J Parasit Dis. 2022. PMID: 36457769 Free PMC article. Review.
-
Slide-and-exchange mechanism for rapid and selective transport through the nuclear pore complex.Proc Natl Acad Sci U S A. 2016 May 3;113(18):E2489-97. doi: 10.1073/pnas.1522663113. Epub 2016 Apr 18. Proc Natl Acad Sci U S A. 2016. PMID: 27091992 Free PMC article.
-
Atypical nuclear envelope condensates linked to neurological disorders reveal nucleoporin-directed chaperone activities.Nat Cell Biol. 2022 Nov;24(11):1630-1641. doi: 10.1038/s41556-022-01001-y. Epub 2022 Oct 27. Nat Cell Biol. 2022. PMID: 36302970 Free PMC article.
-
RanBP2/Nup358 provides a major binding site for NXF1-p15 dimers at the nuclear pore complex and functions in nuclear mRNA export.Mol Cell Biol. 2004 Feb;24(3):1155-67. doi: 10.1128/MCB.24.3.1155-1167.2004. Mol Cell Biol. 2004. PMID: 14729961 Free PMC article.
References
-
- Bayliss R., Ribbeck,K., Akin,D., Kent,H.M., Feldherr,C.M., Görlich,D. and Stewart,M. (1999) Interaction between NTF2 and xFxFG-containing nucleoporins is required to mediate nuclear import of RanGDP. J. Mol. Biol., 293, 579–593. - PubMed
Publication types
MeSH terms
Substances
LinkOut - more resources
Full Text Sources