Detection of direct binding of human herpesvirus 8-encoded interleukin-6 (vIL-6) to both gp130 and IL-6 receptor (IL-6R) and identification of amino acid residues of vIL-6 important for IL-6R-dependent and -independent signaling
- PMID: 11238858
- PMCID: PMC114125
- DOI: 10.1128/JVI.75.7.3325-3334.2001
Detection of direct binding of human herpesvirus 8-encoded interleukin-6 (vIL-6) to both gp130 and IL-6 receptor (IL-6R) and identification of amino acid residues of vIL-6 important for IL-6R-dependent and -independent signaling
Abstract
Human herpesvirus 8 (HHV-8) is associated with Kaposi's sarcoma, primary effusion lymphoma, and multicentric Castleman's disease; in all of these diseases, interleukin-6 (IL-6) has been implicated as a likely mitogenic and/or angiogenic factor. HHV-8 encodes a homologue of IL-6 (viral IL-6 [vIL-6]) that has been shown to be biologically active in several assays and whose activities mirror those of its mammalian counterparts. Like these proteins, vIL-6 mediates its effects through the gp130 signal transducer, but signaling is not dependent on the structurally related IL-6 receptor (IL-6R; gp80) subunit of the receptor-signal transducer complex. However, as we have shown previously, IL-6R can enhance vIL-6 signal transduction and can enable signaling through a gp130 variant (gp130.PM5) that is itself unable to support vIL-6 activity, indicating that IL-6R can form part of the signaling complex. Also, our analysis of a panel of vIL-6 mutants in transfection experiments in Hep3B cells (that express IL-6R and gp130) showed that most were able to function normally in this system. Here, we have used in vitro vIL-6-receptor binding assays to demonstrate direct binding of vIL-6 to both gp130 and IL-6R and vIL-6-induced gp130-IL-6R complex formation, and we have extended our functional analyses of the vIL-6 variants to identify residues important for IL-6R-independent and IL-6R-dependent signaling through native gp130 and gp130.PM5, respectively. These studies have identified residues in vIL-6 that are important for IL-6R-independent and IL-6R-mediated functional complex formation between vIL-6 and gp130 and that may be involved directly in binding to gp130 and IL-6R.
Figures
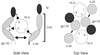
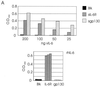
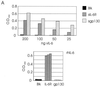
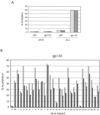
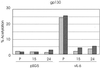
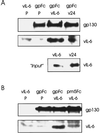
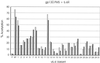
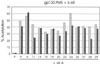
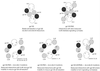
Similar articles
-
Identification of amino acid residues of gp130 signal transducer and gp80 alpha receptor subunit that are involved in ligand binding and signaling by human herpesvirus 8-encoded interleukin-6.J Virol. 2002 Jun;76(11):5627-36. doi: 10.1128/jvi.76.11.5627-5636.2002. J Virol. 2002. PMID: 11991991 Free PMC article.
-
Human herpesvirus 8 interleukin-6 (vIL-6) signals through gp130 but has structural and receptor-binding properties distinct from those of human IL-6.J Virol. 1999 Oct;73(10):8268-78. doi: 10.1128/JVI.73.10.8268-8278.1999. J Virol. 1999. PMID: 10482577 Free PMC article.
-
Molecular mechanisms for viral mimicry of a human cytokine: activation of gp130 by HHV-8 interleukin-6.J Mol Biol. 2004 Jan 9;335(2):641-54. doi: 10.1016/j.jmb.2003.10.070. J Mol Biol. 2004. PMID: 14672670
-
Viral Interleukin-6: Structure, pathophysiology and strategies of neutralization.Eur J Cell Biol. 2011 Jun-Jul;90(6-7):495-504. doi: 10.1016/j.ejcb.2010.10.016. Epub 2010 Dec 21. Eur J Cell Biol. 2011. PMID: 21176991 Review.
-
IL-6/IL-6 receptor system and its role in physiological and pathological conditions.Clin Sci (Lond). 2012 Feb;122(4):143-59. doi: 10.1042/CS20110340. Clin Sci (Lond). 2012. PMID: 22029668 Review.
Cited by
-
The KSHV viral IL-6 homolog is sufficient to induce blood to lymphatic endothelial cell differentiation.Virology. 2012 Jul 5;428(2):112-20. doi: 10.1016/j.virol.2012.03.013. Epub 2012 Apr 21. Virology. 2012. PMID: 22521915 Free PMC article.
-
Genetic Analyses of Contributions of Viral Interleukin-6 Interactions and Signaling to Human Herpesvirus 8 Productive Replication.J Virol. 2020 Sep 15;94(19):e00909-20. doi: 10.1128/JVI.00909-20. Print 2020 Sep 15. J Virol. 2020. PMID: 32669340 Free PMC article.
-
Intracellular signaling mechanisms and activities of human herpesvirus 8 interleukin-6.J Virol. 2009 Jan;83(2):722-33. doi: 10.1128/JVI.01517-08. Epub 2008 Nov 5. J Virol. 2009. PMID: 18987143 Free PMC article.
-
The KSHV viral interleukin-6 is not essential for latency or lytic replication in BJAB cells.Virology. 2007 Mar 15;359(2):425-35. doi: 10.1016/j.virol.2006.09.044. Epub 2006 Oct 30. Virology. 2007. PMID: 17074378 Free PMC article.
-
Human herpesvirus 8 viral interleukin-6 interacts with splice variant 2 of vitamin K epoxide reductase complex subunit 1.J Virol. 2012 Feb;86(3):1577-88. doi: 10.1128/JVI.05782-11. Epub 2011 Nov 30. J Virol. 2012. PMID: 22130532 Free PMC article.
References
-
- Aoki Y, Jaffe E S, Chang Y, Jones K, Teruya-Feldstein J, Moore P S, Tosato G. Angiogenesis and hematopoiesis induced by Kaposi's sarcoma-associated herpesvirus-encoded interleukin-6. Blood. 1999;93:4034–4043. - PubMed
-
- Asou H, Said J W, Yang R, Munker R, Park D J, Kamada N, Koeffler H P. Mechanisms of growth control of Kaposi's sarcoma-associated herpes virus-associated primary effusion lymphoma cells. Blood. 1998;91:2475–2481. - PubMed
-
- Brakenhoff J P J, de Hon F D, Fontaine V, Ten Boekel E, Schooltink H, Rose-John S, Heinrich P C, Content J, Aarden L A. Development of a human interleukin-6 receptor agonist. J Biol Chem. 1994;269:86–93. - PubMed
-
- Burger R, Neipel F, Fleckenstein B, Savino R, Ciliberto G, Kalden J R, Gramatzki G. Human herpesvirus type 8 interleukin-6 homologue is functionally active on human melanoma cell lines. Blood. 1998;91:1858–1863. - PubMed
Publication types
MeSH terms
Substances
Grants and funding
LinkOut - more resources
Full Text Sources
Other Literature Sources
Molecular Biology Databases
Research Materials