Oligomeric structures of poliovirus polymerase are important for function
- PMID: 11230138
- PMCID: PMC145502
- DOI: 10.1093/emboj/20.5.1153
Oligomeric structures of poliovirus polymerase are important for function
Abstract
Central to the replication of poliovirus and other positive-strand RNA viruses is the virally encoded RNA-dependent RNA polymerase. Previous biochemical studies have suggested that direct polymerase- polymerase interactions might be important for polymerase function, and the structure of poliovirus polymerase has revealed two regions of extensive polymerase-polymerase interaction. To explore potential functional roles for the structurally observed polymerase-polymerase interactions, we have performed RNA binding and extension studies of mutant polymerase proteins in solution, disulfide cross-linking studies, mutational analyses in cells, in vitro activity analyses and RNA substrate modeling studies. The results of these studies indicate that both regions of polymerase-polymerase interaction observed in the crystals are indeed functionally important and, furthermore, reveal specific functional roles for each. One of the two regions of interaction provides for efficient substrate RNA binding and the second is crucial for forming catalytic sites. These studies strongly support the hypothesis that the polymerase- polymerase interactions discovered in the crystal structure provide an exquisitely detailed structural context for poliovirus polymerase function and for poliovirus RNA replication in cells.
Figures
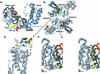
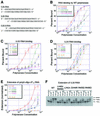
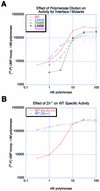
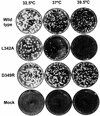
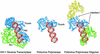
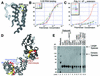
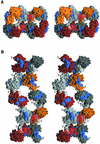
Similar articles
-
Visualization and functional analysis of RNA-dependent RNA polymerase lattices.Science. 2002 Jun 21;296(5576):2218-22. doi: 10.1126/science.1070585. Science. 2002. PMID: 12077417
-
Functional oligomerization of poliovirus RNA-dependent RNA polymerase.RNA. 1995 Jul;1(5):466-77. RNA. 1995. PMID: 7489508 Free PMC article.
-
Studies on the attenuation phenotype of polio vaccines: poliovirus RNA polymerase derived from Sabin type 1 sequence is temperature sensitive in the uridylylation of VPg.Virology. 2000 Jun 20;272(1):72-84. doi: 10.1006/viro.2000.0354. Virology. 2000. PMID: 10873750
-
Analysis of RNA-dependent RNA polymerase structure and function as guided by known polymerase structures and computer predictions of secondary structure.Virology. 1998 Dec 20;252(2):287-303. doi: 10.1006/viro.1998.9463. Virology. 1998. PMID: 9878607 Review.
-
Fidelity of Nucleotide Incorporation by the RNA-Dependent RNA Polymerase from Poliovirus.Enzymes. 2016;39:293-323. doi: 10.1016/bs.enz.2016.02.002. Epub 2016 Mar 28. Enzymes. 2016. PMID: 27241934 Free PMC article. Review.
Cited by
-
Virus factories: biogenesis and structural design.Cell Microbiol. 2013 Jan;15(1):24-34. doi: 10.1111/cmi.12029. Epub 2012 Oct 4. Cell Microbiol. 2013. PMID: 22978691 Free PMC article. Review.
-
Surface for catalysis by poliovirus RNA-dependent RNA polymerase.J Mol Biol. 2013 Jul 24;425(14):2529-40. doi: 10.1016/j.jmb.2013.04.007. Epub 2013 Apr 11. J Mol Biol. 2013. PMID: 23583774 Free PMC article.
-
Biochemical and genetic studies of the initiation of human rhinovirus 2 RNA replication: purification and enzymatic analysis of the RNA-dependent RNA polymerase 3D(pol).J Virol. 2001 Nov;75(22):10969-78. doi: 10.1128/JVI.75.22.10969-10978.2001. J Virol. 2001. PMID: 11602737 Free PMC article.
-
Membrane association of the RNA-dependent RNA polymerase is essential for hepatitis C virus RNA replication.J Virol. 2004 Dec;78(23):13278-84. doi: 10.1128/JVI.78.23.13278-13284.2004. J Virol. 2004. PMID: 15542678 Free PMC article.
-
Initiation of protein-primed picornavirus RNA synthesis.Virus Res. 2015 Aug 3;206:12-26. doi: 10.1016/j.virusres.2014.12.028. Epub 2015 Jan 12. Virus Res. 2015. PMID: 25592245 Free PMC article. Review.
References
-
- Ago H., Adachi,T., Yoshida,A., Yamamoto,M., Habuka,N., Yatsunami,K. and Miyano,M. (1999) Crystal structure of the RNA-dependent RNA polymerase of hepatitis C virus. Struct. Fold. Des., 7, 1417–1426. - PubMed
-
- Arnold J.J. and Cameron,C.E. (1999) Poliovirus RNA-dependent RNA polymerase (3Dpol) is sufficient for template switching in vitro. J. Biol. Chem., 274, 2706–2716. - PubMed
-
- Arnold J.J. and Cameron,C.E. (2000) Poliovirus RNA-dependent RNA polymerase (3D(pol)). Assembly of stable, elongation-competent complexes by using a symmetrical primer-template substrate (sym/sub). J. Biol. Chem., 275, 5329–5336. - PubMed
-
- Beckman M.T.L. and Kirkegaard,K. (1998) Site size of cooperative RNA binding by poliovirus RNA-dependent RNA polymerase. J. Biol. Chem., 273, 6724–6730. - PubMed
Publication types
MeSH terms
Substances
LinkOut - more resources
Full Text Sources
Molecular Biology Databases