Its substrate specificity characterizes the DnaJ co-chaperone as a scanning factor for the DnaK chaperone
- PMID: 11230128
- PMCID: PMC145471
- DOI: 10.1093/emboj/20.5.1042
Its substrate specificity characterizes the DnaJ co-chaperone as a scanning factor for the DnaK chaperone
Abstract
The evolutionarily conserved DnaJ proteins are essential components of Hsp70 chaperone systems. The DnaJ homologue of Escherichia coli associates with chaperone substrates and mediates their ATP hydrolysis-dependent locking into the binding cavity of its Hsp70 partner, DnaK. To determine the substrate specificity of DnaJ proteins, we screened 1633 peptides derived from 14 protein sequences for binding to E.coli DnaJ. The binding motif of DnaJ consists of a hydrophobic core of approximately eight residues enriched for aromatic and large aliphatic hydrophobic residues and arginine. The hydrophobicity of this motif explains why DnaJ itself can prevent protein aggregation. Although this motif shows differences from DnaK's binding motif, DnaJ and DnaK share the majority of binding peptides. In contrast to DnaK, DnaJ binds peptides consisting of L- and D-amino acids, and therefore is not restricted by backbone contacts. These features allow DnaJ to scan hydrophobic protein surfaces and initiate the functional cycle of the DnaK system by associating with hydrophobic exposed patches and subsequent targeting of DnaK to these or to hydrophobic patches in spatial neighbourhood.
Figures
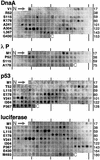
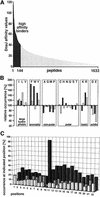
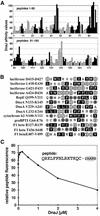
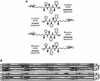
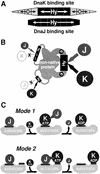
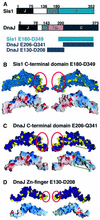
Similar articles
-
Investigation of the interaction between DnaK and DnaJ by surface plasmon resonance spectroscopy.J Mol Biol. 1999 Jun 18;289(4):1131-44. doi: 10.1006/jmbi.1999.2844. J Mol Biol. 1999. PMID: 10369787
-
DnaJ potentiates the interaction between DnaK and alpha-helical peptides.Biochem Biophys Res Commun. 1997 Apr 28;233(3):627-30. doi: 10.1006/bbrc.1997.6454. Biochem Biophys Res Commun. 1997. PMID: 9168902
-
cis-Effect of DnaJ on DnaK in ternary complexes with chimeric DnaK/DnaJ-binding peptides.FEBS Lett. 2004 Apr 9;563(1-3):146-50. doi: 10.1016/S0014-5793(04)00290-X. FEBS Lett. 2004. PMID: 15063739
-
The Escherichia coli chaperones involved in DNA replication.Philos Trans R Soc Lond B Biol Sci. 1993 Mar 29;339(1289):271-7; discussion 277-8. doi: 10.1098/rstb.1993.0025. Philos Trans R Soc Lond B Biol Sci. 1993. PMID: 8098531 Review.
-
Interferon-gamma is a target for binding and folding by both Escherichia coli chaperone model systems GroEL/GroES and DnaK/DnaJ/GrpE.Biochimie. 1998 Aug-Sep;80(8-9):729-37. doi: 10.1016/s0300-9084(99)80026-1. Biochimie. 1998. PMID: 9865495 Review.
Cited by
-
Members of the Hsp70 Family Recognize Distinct Types of Sequences to Execute ER Quality Control.Mol Cell. 2016 Sep 1;63(5):739-52. doi: 10.1016/j.molcel.2016.07.012. Epub 2016 Aug 18. Mol Cell. 2016. PMID: 27546788 Free PMC article.
-
Protein folding in vitro and in the cell: From a solitary journey to a team effort.Biophys Chem. 2022 Aug;287:106821. doi: 10.1016/j.bpc.2022.106821. Epub 2022 Apr 29. Biophys Chem. 2022. PMID: 35667131 Free PMC article. Review.
-
Prion propagation by Hsp40 molecular chaperones.Prion. 2009 Apr-Jun;3(2):59-64. doi: 10.4161/pri.3.2.9062. Epub 2009 Apr 20. Prion. 2009. PMID: 19535913 Free PMC article. Review.
-
Sequence features governing aggregation or degradation of prion-like proteins.PLoS Genet. 2018 Jul 13;14(7):e1007517. doi: 10.1371/journal.pgen.1007517. eCollection 2018 Jul. PLoS Genet. 2018. PMID: 30005071 Free PMC article.
-
The effects of amino acid composition of glutamine-rich domains on amyloid formation and fragmentation.PLoS One. 2012;7(10):e46458. doi: 10.1371/journal.pone.0046458. Epub 2012 Oct 10. PLoS One. 2012. PMID: 23071575 Free PMC article.
References
-
- Banecki B., Liberek,K., Wall,D., Wawrzynów,A., Georgopoulos,C., Bertoli,E., Tanfani,F. and Zylicz,M. (1996) Structure–function analysis of the zinc finger region of the DnaJ molecular chaperone. J. Biol. Chem., 271, 14840–14848. - PubMed
-
- Ellis R.J. and Hartl,F.U. (1999) Principles of protein folding in the cellular environment. Curr. Opin. Struct. Biol., 9, 102–110. - PubMed
-
- Feifel B., Schönfeld,H.-J. and Christen,P. (1998) d-peptide ligands for the co-chaperone DnaJ. J. Biol. Chem., 273, 11999–12002. - PubMed
-
- Frank R. (1992) Spot synthesis: an easy technique for the positionally addressable, parallel chemical synthesis on a membrane support. Tetrahedron, 48, 9217–9232.
Publication types
MeSH terms
Substances
LinkOut - more resources
Full Text Sources
Molecular Biology Databases