A GP64-null baculovirus pseudotyped with vesicular stomatitis virus G protein
- PMID: 11222677
- PMCID: PMC115876
- DOI: 10.1128/JVI.75.6.2544-2556.2001
A GP64-null baculovirus pseudotyped with vesicular stomatitis virus G protein
Abstract
The Autographa californica multiple nucleopolyhedrovirus (AcMNPV) GP64 protein is an essential virion protein that is involved in both receptor binding and membrane fusion during viral entry. Genetic studies have shown that GP64-null viruses are unable to move from cell to cell and this results from a defect in the assembly and production of budded virions (BV). To further examine requirements for virion budding, we asked whether a GP64-null baculovirus, vAc(64-), could be pseudotyped by introducing a heterologous viral envelope protein (vesicular stomatitis virus G protein [VSV-G]) into its membrane and whether the resulting virus was infectious. To address this question, we generated a stably transfected insect Sf9 cell line (Sf9(VSV-G)) that inducibly expresses the VSV-G protein upon infection with AcMNPV Sf9(VSV-G) and Sf9 cells were infected with vAc(64-), and cells were monitored for infection and for movement of infection from cell to cell. vAc(64-) formed plaques on Sf9(VSV-G) cells but not on Sf9 cells, and plaques formed on Sf9(VSV-G) cells were observed only after prolonged intervals. Passage and amplification of vAc(64-) on Sf9(VSV-G) cells resulted in pseudotyped virus particles that contained the VSV-G protein. Cell-to-cell propagation of vAc(64-) in the G-expressing cells was delayed in comparison to wild-type (wt) AcMNPV, and growth curves showed that pseudotyped vAc(64-) was generated at titers of approximately 10(6) to 10(7) infectious units (IU)/ml, compared with titers of approximately 10(8) IU/ml for wt AcMNPV. Propagation and amplification of pseudotyped vAc(64-) virions in Sf9(VSV-G) cells suggests that the VSV-G protein may either possess the signals necessary for baculovirus BV assembly and budding at the cell surface or may otherwise facilitate production of infectious baculovirus virions. The functional complementation of GP64-null viruses by VSV-G protein was further demonstrated by identification of a vAc(64-)-derived virus that had acquired the G gene through recombination with Sf9(VSV-G) cellular DNA. GP64-null viruses expressing the VSV-G gene were capable of productive infection, replication, and propagation in Sf9 cells.
Figures
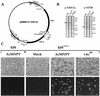

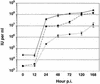
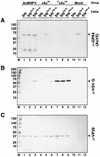
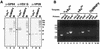
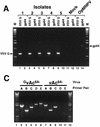
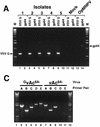
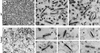
Similar articles
-
Display of heterologous proteins on gp64null baculovirus virions and enhanced budding mediated by a vesicular stomatitis virus G-stem construct.J Virol. 2008 Feb;82(3):1368-77. doi: 10.1128/JVI.02007-07. Epub 2007 Nov 7. J Virol. 2008. PMID: 17989172 Free PMC article.
-
Requirement for GP64 to drive efficient budding of Autographa californica multicapsid nucleopolyhedrovirus.Virology. 1999 Feb 15;254(2):297-314. doi: 10.1006/viro.1998.9523. Virology. 1999. PMID: 9986796
-
Pseudotyping Autographa californica multicapsid nucleopolyhedrovirus (AcMNPV): F proteins from group II NPVs are functionally analogous to AcMNPV GP64.J Virol. 2002 Jun;76(11):5729-36. doi: 10.1128/jvi.76.11.5729-5736.2002. J Virol. 2002. PMID: 11992001 Free PMC article.
-
The GP64 envelope fusion protein is an essential baculovirus protein required for cell-to-cell transmission of infection.J Virol. 1996 Jul;70(7):4607-16. doi: 10.1128/JVI.70.7.4607-4616.1996. J Virol. 1996. PMID: 8676487 Free PMC article.
-
Baculovirus GP64-mediated entry into mammalian cells.J Virol. 2012 Mar;86(5):2610-20. doi: 10.1128/JVI.06704-11. Epub 2011 Dec 21. J Virol. 2012. PMID: 22190715 Free PMC article.
Cited by
-
WSSV ie1 promoter is more efficient than CMV promoter to express H5 hemagglutinin from influenza virus in baculovirus as a chicken vaccine.BMC Microbiol. 2008 Dec 31;8:238. doi: 10.1186/1471-2180-8-238. BMC Microbiol. 2008. PMID: 19116038 Free PMC article.
-
Novel inhibitors of severe acute respiratory syndrome coronavirus entry that act by three distinct mechanisms.J Virol. 2013 Jul;87(14):8017-28. doi: 10.1128/JVI.00998-13. Epub 2013 May 15. J Virol. 2013. PMID: 23678171 Free PMC article.
-
Baculoviruses as Vectors for Gene Therapy against Human Prostate Cancer.J Biomed Biotechnol. 2003;2003(2):79-91. doi: 10.1155/S1110724303209049. J Biomed Biotechnol. 2003. PMID: 12721513 Free PMC article.
-
Baculovirus display: a multifunctional technology for gene delivery and eukaryotic library development.Adv Virus Res. 2006;68:91-112. doi: 10.1016/S0065-3527(06)68003-2. Adv Virus Res. 2006. PMID: 16997010 Free PMC article. Review.
-
Innate immune response induced by baculovirus attenuates transgene expression in mammalian cells.J Virol. 2014 Feb;88(4):2157-67. doi: 10.1128/JVI.03055-13. Epub 2013 Dec 11. J Virol. 2014. PMID: 24335288 Free PMC article.
References
-
- Barsoum J, Brown R, McKee M, Boyce F M. Efficient transduction of mammalian cells by a recombinant baculovirus having the vesicular stomatitis virus G glycoprotein. Hum Gene Ther. 1997;8:2011–2018. - PubMed
-
- Blum H, Beier H, Gross H J. Improved silver staining of plant proteins, RNA and DNA in polyacrylamide gels. Electrophoresis. 1987;8:93–99.
Publication types
MeSH terms
Substances
Grants and funding
LinkOut - more resources
Full Text Sources
Other Literature Sources