Effect of intragenic rearrangement and changes in the 3' consensus sequence on NSP1 expression and rotavirus replication
- PMID: 11160712
- PMCID: PMC114792
- DOI: 10.1128/JVI.75.5.2076-2086.2001
Effect of intragenic rearrangement and changes in the 3' consensus sequence on NSP1 expression and rotavirus replication
Abstract
The nonpolyadenylated mRNAs of rotavirus are templates for the synthesis of protein and the segmented double-stranded RNA (dsRNA) genome. During serial passage of simian SA11 rotaviruses in cell culture, two variants emerged with gene 5 dsRNAs containing large (1.1 and 0.5 kb) sequence duplications within the open reading frame (ORF) for NSP1. Due to the sequence rearrangements, both variants encoded only C-truncated forms of NSP1. Comparison of these and other variants encoding defective NSP1 with their corresponding wild-type viruses indicated that the inability to encode authentic NSP1 results in a small-plaque phenotype. Thus, although nonessential, NSP1 probably plays an active role in rotavirus replication in cell culture. In determining the sequences of the gene 5 dsRNAs of the SA11 variants and wild-type viruses, it was unexpectedly found that their 3' termini ended with 5'-UGAACC-3' instead of the 3' consensus sequence 5'-UGACC-3', which is present on the mRNAs of nearly all other group A rotaviruses. Cell-free assays indicated that the A insertion into the 3' consensus sequence interfered with its ability to promote dsRNA synthesis and to function as a translation enhancer. The results provide evidence that the 3' consensus sequence of the gene 5 dsRNAs of SA11 rotaviruses has undergone a mutation causing it to operate suboptimally in RNA replication and in the expression of NSP1 during the virus life cycle. Indeed, just as rotavirus variants which encode defective NSP1 appear to have a selective advantage over those encoding wild-type NSP1 in cell culture, it may be that the atypical 3' end of SA11 gene 5 has been selected for because it promotes the expression of lower levels of NSP1 than the 3' consensus sequence.
Figures
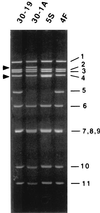
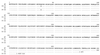
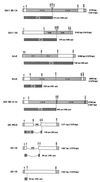
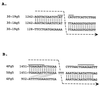
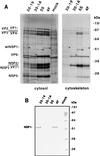
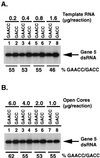
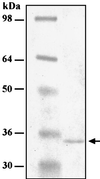
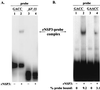
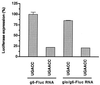
Similar articles
-
Generation of Recombinant Rotavirus Expressing NSP3-UnaG Fusion Protein by a Simplified Reverse Genetics System.J Virol. 2019 Nov 26;93(24):e01616-19. doi: 10.1128/JVI.01616-19. Print 2019 Dec 15. J Virol. 2019. PMID: 31597761 Free PMC article.
-
Cell-line-induced mutation of the rotavirus genome alters expression of an IRF3-interacting protein.EMBO J. 2004 Oct 13;23(20):4072-81. doi: 10.1038/sj.emboj.7600408. Epub 2004 Sep 16. EMBO J. 2004. PMID: 15372078 Free PMC article.
-
Rotavirus NSP1 Contributes to Intestinal Viral Replication, Pathogenesis, and Transmission.mBio. 2021 Dec 21;12(6):e0320821. doi: 10.1128/mBio.03208-21. Epub 2021 Dec 14. mBio. 2021. PMID: 34903043 Free PMC article.
-
Structure and function of rotavirus NSP1.Arch Virol Suppl. 1996;12:53-8. doi: 10.1007/978-3-7091-6553-9_6. Arch Virol Suppl. 1996. PMID: 9015101 Review.
-
Rotavirus RNA replication and gene expression.Novartis Found Symp. 2001;238:64-77; discussion 77-81. doi: 10.1002/0470846534.ch5. Novartis Found Symp. 2001. PMID: 11444036 Review.
Cited by
-
Generation of genetically stable recombinant rotaviruses containing novel genome rearrangements and heterologous sequences by reverse genetics.J Virol. 2013 Jun;87(11):6211-20. doi: 10.1128/JVI.00413-13. Epub 2013 Mar 27. J Virol. 2013. PMID: 23536662 Free PMC article.
-
Recent advances in rotavirus reverse genetics and its utilization in basic research and vaccine development.Arch Virol. 2021 Sep;166(9):2369-2386. doi: 10.1007/s00705-021-05142-7. Epub 2021 Jul 3. Arch Virol. 2021. PMID: 34216267 Free PMC article. Review.
-
Nontemplated terminal nucleotidyltransferase activity of double-stranded RNA bacteriophage phi6 RNA-dependent RNA polymerase.J Virol. 2008 Sep;82(18):9254-64. doi: 10.1128/JVI.01044-08. Epub 2008 Jul 9. J Virol. 2008. PMID: 18614640 Free PMC article.
-
Rotavirus antagonism of the innate immune response.Viruses. 2009 Dec;1(3):1035-56. doi: 10.3390/v1031035. Epub 2009 Nov 24. Viruses. 2009. PMID: 21994581 Free PMC article.
-
Generation of Recombinant Rotaviruses Expressing Fluorescent Proteins by Using an Optimized Reverse Genetics System.J Virol. 2018 Jun 13;92(13):e00588-18. doi: 10.1128/JVI.00588-18. Print 2018 Jul 1. J Virol. 2018. PMID: 29669834 Free PMC article.
References
-
- Chnaiderman J, Diaz J, Magnusson G, Liprandi F, Spencer E. Characterization of a rotavirus rearranged gene 11 by gene reassortment. Arch Virol. 1998;143:1711–1722. - PubMed
Publication types
MeSH terms
Substances
Associated data
- Actions
- Actions
- Actions
- Actions
LinkOut - more resources
Full Text Sources