Transmembrane transforming growth factor-alpha tethers to the PDZ domain-containing, Golgi membrane-associated protein p59/GRASP55
- PMID: 11101516
- PMCID: PMC305863
- DOI: 10.1093/emboj/19.23.6427
Transmembrane transforming growth factor-alpha tethers to the PDZ domain-containing, Golgi membrane-associated protein p59/GRASP55
Abstract
Transforming growth factor-alpha (TGF-alpha) and related proteins represent a family of transmembrane growth factors with representatives in flies and worms. Little is known about the transport of TGF-alpha and other transmembrane growth factors to the cell surface and its regulation. p59 was purified as a cytoplasmic protein, which at endogenous levels associates with transmembrane TGF-alpha. cDNA cloning of p59 revealed a 452 amino acid sequence with two PDZ domains. p59 is myristoylated and palmitoylated, and associates with the Golgi system, where it co-localizes with TGF-alpha. Its first PDZ domain interacts with the C-terminus of transmembrane TGF-alpha and select transmembrane proteins. p59 is the human homolog of GRASP55, which is structurally related to GRASP65. GRASP55 and GRASP65 have been shown to play a role in stacking of the Golgi cisternae in vitro. C-terminal mutations of transmembrane TGF-alpha, which decrease or abolish the interaction with p59, also strongly impair cell surface expression of TGF-alpha. Our observations suggest a role for membrane tethering of p59/GRASP55 to select transmembrane proteins, including TGF-alpha, in maturation and transport to the cell surface.
Figures
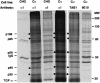
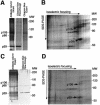
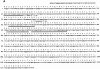
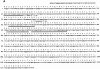
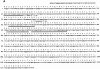
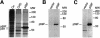
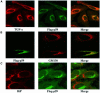
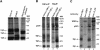
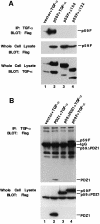
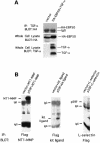
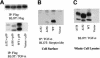
Similar articles
-
GRASP55, a second mammalian GRASP protein involved in the stacking of Golgi cisternae in a cell-free system.EMBO J. 1999 Sep 15;18(18):4949-60. doi: 10.1093/emboj/18.18.4949. EMBO J. 1999. PMID: 10487747 Free PMC article.
-
Identification and characterization of a novel Golgi protein, GCP60, that interacts with the integral membrane protein giantin.J Biol Chem. 2001 Nov 30;276(48):45298-306. doi: 10.1074/jbc.M108961200. Epub 2001 Oct 5. J Biol Chem. 2001. PMID: 11590181
-
Structural Basis for the Interaction between Golgi Reassembly-stacking Protein GRASP55 and Golgin45.J Biol Chem. 2017 Feb 17;292(7):2956-2965. doi: 10.1074/jbc.M116.765990. Epub 2017 Jan 3. J Biol Chem. 2017. PMID: 28049725 Free PMC article.
-
GRASP55: A Multifunctional Protein.Curr Protein Pept Sci. 2020;21(6):544-552. doi: 10.2174/1389203721666200218105302. Curr Protein Pept Sci. 2020. PMID: 32067616 Review.
-
Protein palmitoylation in membrane trafficking.Biochem Soc Trans. 1995 Aug;23(3):572-6. doi: 10.1042/bst0230572. Biochem Soc Trans. 1995. PMID: 8566419 Review. No abstract available.
Cited by
-
The cytoplasmic domain of proEGF negatively regulates motility and elastinolytic activity in thyroid carcinoma cells.Neoplasia. 2008 Oct;10(10):1120-30. doi: 10.1593/neo.08580. Neoplasia. 2008. PMID: 18813355 Free PMC article.
-
Genetic, structural, and chemical insights into the dual function of GRASP55 in germ cell Golgi remodeling and JAM-C polarized localization during spermatogenesis.PLoS Genet. 2017 Jun 15;13(6):e1006803. doi: 10.1371/journal.pgen.1006803. eCollection 2017 Jun. PLoS Genet. 2017. PMID: 28617811 Free PMC article.
-
The role of protease activity in ErbB biology.Exp Cell Res. 2009 Feb 15;315(4):671-82. doi: 10.1016/j.yexcr.2008.10.011. Epub 2008 Oct 25. Exp Cell Res. 2009. PMID: 19013149 Free PMC article. Review.
-
GRASP65 and GRASP55 sequentially promote the transport of C-terminal valine-bearing cargos to and through the Golgi complex.J Biol Chem. 2009 Dec 11;284(50):34849-60. doi: 10.1074/jbc.M109.068403. Epub 2009 Oct 19. J Biol Chem. 2009. PMID: 19840934 Free PMC article.
-
A direct role for GRASP65 as a mitotically regulated Golgi stacking factor.EMBO J. 2003 Jul 1;22(13):3279-90. doi: 10.1093/emboj/cdg317. EMBO J. 2003. PMID: 12839990 Free PMC article.
References
-
- Barr F.A., Puype,M., Vandekerckhove,J. and Warren,G. (1997) GRASP65, a protein involved in the stacking of Golgi cisternae. Cell, 91, 253–262. - PubMed
-
- Baselga J., Mendelsohn,J., Kim,Y.M. and Pandiella,A. (1996) Autocrine regulation of transforming growth factor-α cleavage. J. Biol. Chem., 271, 3279–3284. - PubMed
-
- Bischoff J., Liscum,L. and Kornfeld,R. (1986) The use of deoxy mannojirimycin to evaluate the role of various α-mannosidases in oligosaccharide processing in intact cells. J. Biol. Chem., 261, 4766–4774. - PubMed
Publication types
MeSH terms
Substances
Grants and funding
LinkOut - more resources
Full Text Sources
Molecular Biology Databases