The AP-3 complex required for endosomal synaptic vesicle biogenesis is associated with a casein kinase Ialpha-like isoform
- PMID: 10930456
- PMCID: PMC14942
- DOI: 10.1091/mbc.11.8.2591
The AP-3 complex required for endosomal synaptic vesicle biogenesis is associated with a casein kinase Ialpha-like isoform
Abstract
The formation of small vesicles is mediated by cytoplasmic coats the assembly of which is regulated by the activity of GTPases, kinases, and phosphatases. A heterotetrameric AP-3 adaptor complex has been implicated in the formation of synaptic vesicles from PC12 endosomes (). When the small GTPase ARF1 is prevented from hydrolyzing GTP, we can reconstitute AP-3 recruitment to synaptic vesicle membranes in an assembly reaction that requires temperatures above 15 degrees C and the presence of ATP suggesting that an enzymatic step is involved in the coat assembly. We have now found an enzymatic reaction, the phosphorylation of the AP-3 adaptor complex, that is linked with synaptic vesicle coating. Phosphorylation occurs in the beta3 subunit of the complex by a kinase similar to casein kinase 1alpha. The kinase copurifies with neuronal-specific AP-3. In vitro, purified casein kinase I selectively phosphorylates the beta3A and beta3B subunit at its hinge domain. Inhibiting the kinase hinders the recruitment of AP-3 to synaptic vesicles. The same inhibitors that prevent coat assembly in vitro also inhibit the formation of synaptic vesicles in PC12 cells. The data suggest, therefore, that the mechanism of AP-3-mediated vesiculation from neuroendocrine endosomes requires the phosphorylation of the adaptor complex at a step during or after AP-3 recruitment to membranes.
Figures
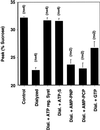
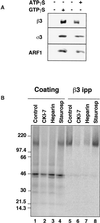
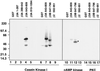
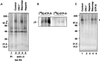
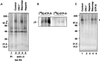
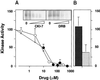
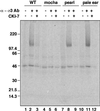
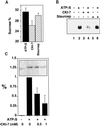
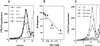
Similar articles
-
A function for the AP3 coat complex in synaptic vesicle formation from endosomes.Cell. 1998 May 1;93(3):423-32. doi: 10.1016/s0092-8674(00)81170-8. Cell. 1998. PMID: 9590176
-
The neuronal form of adaptor protein-3 is required for synaptic vesicle formation from endosomes.J Neurosci. 2001 Oct 15;21(20):8034-42. doi: 10.1523/JNEUROSCI.21-20-08034.2001. J Neurosci. 2001. PMID: 11588176 Free PMC article.
-
A v-SNARE participates in synaptic vesicle formation mediated by the AP3 adaptor complex.Nat Neurosci. 1998 Nov;1(7):551-6. doi: 10.1038/2787. Nat Neurosci. 1998. PMID: 10196561
-
Clathrin and adaptors.Biochim Biophys Acta. 1998 Aug 14;1404(1-2):173-93. doi: 10.1016/s0167-4889(98)00056-1. Biochim Biophys Acta. 1998. PMID: 9714795 Review.
-
Mechanisms of protein sorting and coat assembly: insights from the clathrin-coated vesicle pathway.Curr Opin Cell Biol. 1998 Aug;10(4):499-503. doi: 10.1016/s0955-0674(98)80065-3. Curr Opin Cell Biol. 1998. PMID: 9719871 Review.
Cited by
-
Variation in PTCHD2, CRISP3, NAP1L4, FSCB, and AP3B2 associated with spherical equivalent.Mol Vis. 2016 Jul 14;22:783-96. eCollection 2016. Mol Vis. 2016. PMID: 27440996 Free PMC article.
-
LRRK2 and RAB7L1 coordinately regulate axonal morphology and lysosome integrity in diverse cellular contexts.Sci Rep. 2016 Jul 18;6:29945. doi: 10.1038/srep29945. Sci Rep. 2016. PMID: 27424887 Free PMC article.
-
Inositol pyrophosphate mediated pyrophosphorylation of AP3B1 regulates HIV-1 Gag release.Proc Natl Acad Sci U S A. 2009 Dec 15;106(50):21161-6. doi: 10.1073/pnas.0909176106. Epub 2009 Nov 23. Proc Natl Acad Sci U S A. 2009. PMID: 19934039 Free PMC article.
-
A molecular genetics laboratory course applying bioinformatics and cell biology in the context of original research.CBE Life Sci Educ. 2008 Winter;7(4):410-21. doi: 10.1187/cbe.08-07-0036. CBE Life Sci Educ. 2008. PMID: 19047427 Free PMC article.
-
Vesicular zinc regulates the Ca2+ sensitivity of a subpopulation of presynaptic vesicles at hippocampal mossy fiber terminals.J Neurosci. 2011 Dec 14;31(50):18251-65. doi: 10.1523/JNEUROSCI.4164-11.2011. J Neurosci. 2011. PMID: 22171030 Free PMC article.
References
-
- Anderson HA, Roche PA. Phosphorylation regulates the delivery of MHC class II invariant chain complexes to antigen processing compartments. J Immunol. 1998;160:4850–4858. - PubMed
-
- Bauerfeind R, Takei K, De Camilli P. Amphiphysin I is associated with coated endocytic intermediates and undergoes stimulation-dependent dephosphorylation in nerve terminals. J Biol Chem. 1997;272:30984–30992. - PubMed
-
- Beyaert R, Vanhaesebroeck B, Declercq W, Van Lint J, Vandenabele P, Agostinis P, Vandenheede JR, Fiers W. Casein kinase-1 phosphorylates the p75 tumor necrosis factor receptor and negatively regulates tumor necrosis factor signaling for apoptosis. J Biol Chem. 1995;270:23293–23299. - PubMed
-
- Bioukar EB, Marricco NC, Zuo D, Larose L. Serine phosphorylation of the ligand-activated beta-platelet-derived growth factor receptor by casein kinase I-gamma2 inhibits the receptor's autophosphorylating activity. J Biol Chem. 1999;274:21457–21463. - PubMed
Publication types
MeSH terms
Substances
Grants and funding
LinkOut - more resources
Full Text Sources
Molecular Biology Databases