Relief of two built-In autoinhibitory mechanisms in P-TEFb is required for assembly of a multicomponent transcription elongation complex at the human immunodeficiency virus type 1 promoter
- PMID: 10913173
- PMCID: PMC86067
- DOI: 10.1128/MCB.20.16.5897-5907.2000
Relief of two built-In autoinhibitory mechanisms in P-TEFb is required for assembly of a multicomponent transcription elongation complex at the human immunodeficiency virus type 1 promoter
Abstract
Tat stimulation of human immunodeficiency virus type 1 (HIV-1) transcription requires Tat-dependent recruitment of human positive transcription elongation factor b (P-TEFb) to the HIV-1 promoter and the formation on the trans-acting response element (TAR) RNA of a P-TEFb-Tat-TAR ternary complex. We show here that the P-TEFb heterodimer of Cdk9-cyclin T1 is intrinsically incapable of forming a stable complex with Tat and TAR due to two built-in autoinhibitory mechanisms in P-TEFb. Both mechanisms exert little effect on the P-TEFb-Tat interaction but prevent the P-TEFb-Tat complex from binding to TAR RNA. The first autoinhibition arises from the unphosphorylated state of Cdk9, which establishes a P-TEFb conformation unfavorable for TAR recognition. Autophosphorylation of Cdk9 overcomes this inhibition by inducing conformational changes in P-TEFb, thereby exposing a region in cyclin T1 for possible TAR binding. An intramolecular interaction between the N- and C-terminal regions of cyclin T1 sterically blocks the P-TEFb-TAR interaction and constitutes the second autoinhibitory mechanism. This inhibition is relieved by the binding of the C-terminal region of cyclin T1 to the transcription elongation factor Tat-SF1 and perhaps other cellular factors. Upon release from the intramolecular interaction, the C-terminal region also interacts with RNA polymerase II and is required for HIV-1 transcription, suggesting its role in bridging the P-TEFb-Tat-TAR complex and the basal elongation apparatus. These data reveal novel control mechanisms for the assembly of a multicomponent transcription elongation complex at the HIV-1 promoter.
Figures
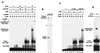
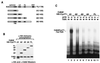
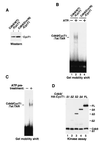
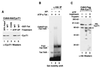
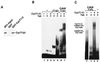
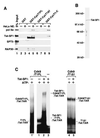
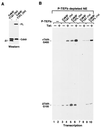
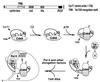
Similar articles
-
CDK9 autophosphorylation regulates high-affinity binding of the human immunodeficiency virus type 1 tat-P-TEFb complex to TAR RNA.Mol Cell Biol. 2000 Sep;20(18):6958-69. doi: 10.1128/MCB.20.18.6958-6969.2000. Mol Cell Biol. 2000. PMID: 10958691 Free PMC article.
-
Specific interaction of Tat with the human but not rodent P-TEFb complex mediates the species-specific Tat activation of HIV-1 transcription.Proc Natl Acad Sci U S A. 1999 Mar 16;96(6):2728-33. doi: 10.1073/pnas.96.6.2728. Proc Natl Acad Sci U S A. 1999. PMID: 10077579 Free PMC article.
-
An in vitro transcription system that recapitulates equine infectious anemia virus tat-mediated inhibition of human immunodeficiency virus type 1 Tat activity demonstrates a role for positive transcription elongation factor b and associated proteins in the mechanism of Tat activation.Virology. 2000 Sep 1;274(2):356-66. doi: 10.1006/viro.2000.0480. Virology. 2000. PMID: 10964778
-
Tackling Tat.J Mol Biol. 1999 Oct 22;293(2):235-54. doi: 10.1006/jmbi.1999.3060. J Mol Biol. 1999. PMID: 10550206 Review.
-
Regulatory functions of Cdk9 and of cyclin T1 in HIV tat transactivation pathway gene expression.J Cell Biochem. 1999 Dec 1;75(3):357-68. J Cell Biochem. 1999. PMID: 10536359 Review.
Cited by
-
CDK9: A Comprehensive Review of Its Biology, and Its Role as a Potential Target for Anti-Cancer Agents.Front Oncol. 2021 May 10;11:678559. doi: 10.3389/fonc.2021.678559. eCollection 2021. Front Oncol. 2021. PMID: 34041038 Free PMC article. Review.
-
RNA polymerase II elongation control.Annu Rev Biochem. 2012;81:119-43. doi: 10.1146/annurev-biochem-052610-095910. Epub 2012 Mar 9. Annu Rev Biochem. 2012. PMID: 22404626 Free PMC article. Review.
-
The pre-mRNA splicing and transcription factor Tat-SF1 is a functional partner of the spliceosome SF3b1 subunit via a U2AF homology motif interface.J Biol Chem. 2019 Feb 22;294(8):2892-2902. doi: 10.1074/jbc.RA118.006764. Epub 2018 Dec 19. J Biol Chem. 2019. PMID: 30567737 Free PMC article.
-
G-actin participates in RNA polymerase II-dependent transcription elongation by recruiting positive transcription elongation factor b (P-TEFb).J Biol Chem. 2011 Apr 29;286(17):15171-81. doi: 10.1074/jbc.M110.184374. Epub 2011 Mar 4. J Biol Chem. 2011. PMID: 21378166 Free PMC article.
-
Acetylation of cyclin T1 regulates the equilibrium between active and inactive P-TEFb in cells.EMBO J. 2009 May 20;28(10):1407-17. doi: 10.1038/emboj.2009.99. Epub 2009 Apr 23. EMBO J. 2009. PMID: 19387490 Free PMC article.
References
-
- Chun R F, Jeang K T. Requirements for RNA polymerase II carboxyl-terminal domain for activated transcription of human retroviruses human T-cell lymphotropic virus I and HIV-1. J Biol Chem. 1996;271:27888–27894. - PubMed
Publication types
MeSH terms
Substances
Grants and funding
LinkOut - more resources
Full Text Sources
Molecular Biology Databases
Miscellaneous