Characterization of the vaccinia virus H3L envelope protein: topology and posttranslational membrane insertion via the C-terminal hydrophobic tail
- PMID: 10906204
- PMCID: PMC112271
- DOI: 10.1128/jvi.74.16.7508-7517.2000
Characterization of the vaccinia virus H3L envelope protein: topology and posttranslational membrane insertion via the C-terminal hydrophobic tail
Abstract
The vaccinia virus H3L open reading frame encodes a 324-amino-acid immunodominant membrane component of virus particles. Biochemical and microscopic studies demonstrated that the H3L protein was expressed late in infection, accumulated in the cytoplasmic viral factory regions, and associated primarily with amorphous material near immature virions and with intracellular virion membranes. Localization of the H3L protein on the surfaces of viral particles and anchorage via the hydrophobic tail were consistent with its extraction by NP-40 in the absence of reducing agents, its trypsin sensitivity, its reactivity with a membrane-impermeable biotinylation reagent, and its immunogold labeling with an antibody to a peptide comprising amino acids 247 to 259. The H3L protein, synthesized in a coupled in vitro transcription/translation system, was tightly anchored to membranes as determined by resistance to Na(2)CO(3) (pH 11) extraction and cytoplasmically oriented as shown by sensitivity to proteinase K digestion. Further studies demonstrated that membrane insertion of the H3L protein occurred posttranslationally and that the C-terminal hydrophobic domain was necessary and sufficient for this to occur. These data indicated that the H3L protein is a member of the C-terminal anchor family and supported a model in which it is synthesized on free ribosomes and inserts into the membranes of viral particles during their maturation.
Figures
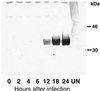
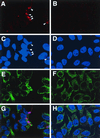
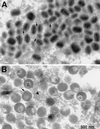
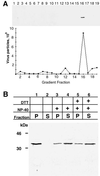
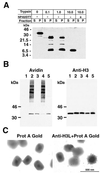
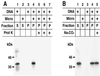
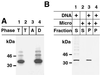
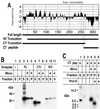
Similar articles
-
Effects of deletion or stringent repression of the H3L envelope gene on vaccinia virus replication.J Virol. 2000 Aug;74(16):7518-28. doi: 10.1128/jvi.74.16.7518-7528.2000. J Virol. 2000. PMID: 10906205 Free PMC article.
-
Vaccinia virus envelope H3L protein binds to cell surface heparan sulfate and is important for intracellular mature virion morphogenesis and virus infection in vitro and in vivo.J Virol. 2000 Apr;74(7):3353-65. doi: 10.1128/jvi.74.7.3353-3365.2000. J Virol. 2000. PMID: 10708453 Free PMC article.
-
Vaccinia virus G7L protein Interacts with the A30L protein and is required for association of viral membranes with dense viroplasm to form immature virions.J Virol. 2003 Mar;77(6):3418-29. doi: 10.1128/jvi.77.6.3418-3429.2003. J Virol. 2003. PMID: 12610117 Free PMC article.
-
In a nutshell: structure and assembly of the vaccinia virion.Adv Virus Res. 2006;66:31-124. doi: 10.1016/S0065-3527(06)66002-8. Adv Virus Res. 2006. PMID: 16877059 Review.
-
Coronavirus envelope protein: a small membrane protein with multiple functions.Cell Mol Life Sci. 2007 Aug;64(16):2043-8. doi: 10.1007/s00018-007-7103-1. Cell Mol Life Sci. 2007. PMID: 17530462 Free PMC article. Review.
Cited by
-
Analysis of the monkeypox virus genome.Virology. 2002 Jun 5;297(2):172-94. doi: 10.1006/viro.2002.1446. Virology. 2002. PMID: 12083817 Free PMC article.
-
Synthesis and function of membrane phosphoinositides in budding yeast, Saccharomyces cerevisiae.Biochim Biophys Acta. 2007 Mar;1771(3):353-404. doi: 10.1016/j.bbalip.2007.01.015. Epub 2007 Feb 6. Biochim Biophys Acta. 2007. PMID: 17382260 Free PMC article. Review.
-
Repression of vaccinia virus Holliday junction resolvase inhibits processing of viral DNA into unit-length genomes.J Virol. 2001 Jul;75(14):6460-71. doi: 10.1128/JVI.75.14.6460-6471.2001. J Virol. 2001. PMID: 11413313 Free PMC article.
-
Virulence in murine model shows the existence of two distinct populations of Brazilian Vaccinia virus strains.PLoS One. 2008 Aug 26;3(8):e3043. doi: 10.1371/journal.pone.0003043. PLoS One. 2008. PMID: 18725979 Free PMC article.
-
Redistribution of cyclophilin A to viral factories during vaccinia virus infection and its incorporation into mature particles.J Virol. 2003 Aug;77(16):9052-68. doi: 10.1128/jvi.77.16.9052-9068.2003. J Virol. 2003. PMID: 12885921 Free PMC article.
References
Publication types
MeSH terms
Substances
LinkOut - more resources
Full Text Sources
Other Literature Sources
Miscellaneous