Uracil-DNA glycosylase-DNA substrate and product structures: conformational strain promotes catalytic efficiency by coupled stereoelectronic effects
- PMID: 10805771
- PMCID: PMC25785
- DOI: 10.1073/pnas.97.10.5083
Uracil-DNA glycosylase-DNA substrate and product structures: conformational strain promotes catalytic efficiency by coupled stereoelectronic effects
Abstract
Enzymatic transformations of macromolecular substrates such as DNA repair enzyme/DNA transformations are commonly interpreted primarily by active-site functional-group chemistry that ignores their extensive interfaces. Yet human uracil-DNA glycosylase (UDG), an archetypical enzyme that initiates DNA base-excision repair, efficiently excises the damaged base uracil resulting from cytosine deamination even when active-site functional groups are deleted by mutagenesis. The 1.8-A resolution substrate analogue and 2.0-A resolution cleaved product cocrystal structures of UDG bound to double-stranded DNA suggest enzyme-DNA substrate-binding energy from the macromolecular interface is funneled into catalytic power at the active site. The architecturally stabilized closing of UDG enforces distortions of the uracil and deoxyribose in the flipped-out nucleotide substrate that are relieved by glycosylic bond cleavage in the product complex. This experimentally defined substrate stereochemistry implies the enzyme alters the orientation of three orthogonal electron orbitals to favor electron transpositions for glycosylic bond cleavage. By revealing the coupling of this anomeric effect to a delocalization of the glycosylic bond electrons into the uracil aromatic system, this structurally implicated mechanism resolves apparent paradoxes concerning the transpositions of electrons among orthogonal orbitals and the retention of catalytic efficiency despite mutational removal of active-site functional groups. These UDG/DNA structures and their implied dissociative excision chemistry suggest biology favors a chemistry for base-excision repair initiation that optimizes pathway coordination by product binding to avoid the release of cytotoxic and mutagenic intermediates. Similar excision chemistry may apply to other biological reaction pathways requiring the coordination of complex multistep chemical transformations.
Figures
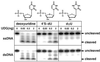
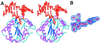
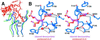
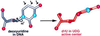
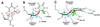
Similar articles
-
Base excision repair initiation revealed by crystal structures and binding kinetics of human uracil-DNA glycosylase with DNA.EMBO J. 1998 Sep 1;17(17):5214-26. doi: 10.1093/emboj/17.17.5214. EMBO J. 1998. PMID: 9724657 Free PMC article.
-
Crystal structure of Escherichia coli uracil DNA glycosylase and its complexes with uracil and glycerol: structure and glycosylase mechanism revisited.Proteins. 1999 Apr 1;35(1):13-24. Proteins. 1999. PMID: 10090282
-
A nucleotide-flipping mechanism from the structure of human uracil-DNA glycosylase bound to DNA.Nature. 1996 Nov 7;384(6604):87-92. doi: 10.1038/384087a0. Nature. 1996. PMID: 8900285
-
DNA glycosylases in the base excision repair of DNA.Biochem J. 1997 Jul 1;325 ( Pt 1)(Pt 1):1-16. doi: 10.1042/bj3250001. Biochem J. 1997. PMID: 9224623 Free PMC article. Review.
-
Lessons learned from structural results on uracil-DNA glycosylase.Mutat Res. 2000 Aug 30;460(3-4):183-99. doi: 10.1016/s0921-8777(00)00026-4. Mutat Res. 2000. PMID: 10946228 Review.
Cited by
-
Expanding targets of DNAzyme-based sensors through deactivation and activation of DNAzymes by single uracil removal: sensitive fluorescent assay of uracil-DNA glycosylase.Anal Chem. 2012 Nov 20;84(22):9981-7. doi: 10.1021/ac302424f. Epub 2012 Oct 29. Anal Chem. 2012. PMID: 23072386 Free PMC article.
-
Comparison of gas phase intrinsic properties of cytosine and thymine nucleobases with their O-alkyl adducts: different hydrogen bonding preferences for thymine versus O-alkyl thymine.J Mol Model. 2013 Aug;19(8):2993-3005. doi: 10.1007/s00894-013-1813-0. Epub 2013 Apr 7. J Mol Model. 2013. PMID: 23564326
-
An effective human uracil-DNA glycosylase inhibitor targets the open pre-catalytic active site conformation.Prog Biophys Mol Biol. 2021 Aug;163:143-159. doi: 10.1016/j.pbiomolbio.2021.02.004. Epub 2021 Mar 3. Prog Biophys Mol Biol. 2021. PMID: 33675849 Free PMC article.
-
Structural Basis for Excision of 5-Formylcytosine by Thymine DNA Glycosylase.Biochemistry. 2016 Nov 15;55(45):6205-6208. doi: 10.1021/acs.biochem.6b00982. Epub 2016 Nov 2. Biochemistry. 2016. PMID: 27805810 Free PMC article.
-
Sequence-dependent base pair opening in DNA double helix.Biophys J. 2006 May 1;90(9):3091-9. doi: 10.1529/biophysj.105.078774. Epub 2006 Feb 24. Biophys J. 2006. PMID: 16500982 Free PMC article.
References
-
- Davies G J, Mackenzie L, Varrot A, Dauter M, Brzozowski A M, Schulein M, Withers S G. Biochemistry. 1998;37:11707–11713. - PubMed
-
- Sidhu G, Withers S G, Nguyen N T, McIntosh L P, Ziser L, Brayer G D. Biochemistry. 1999;38:5346–5354. - PubMed
-
- Uitdehaag J C, Mosi R, Kalk K H, vanderVeen B A, Dijkhuizen L, Withers S G, Dijkstra B W. Nat Struct Biol. 1999;6:432–436. - PubMed
-
- Fersht A. Structure and Mechanism in Protein Science: A Guide to Enzyme Catalysis and Protein Folding. New York: Freeman; 1999.
-
- Kirby A J. The Anomeric Effect and Related Stereoelectronic Effects at Oxygen. Berlin: Springer; 1983.
Publication types
MeSH terms
Substances
Associated data
- Actions
- Actions
LinkOut - more resources
Full Text Sources
Other Literature Sources
Molecular Biology Databases
Research Materials