Structural requirements for ligand binding by a probable plant vacuolar sorting receptor
- PMID: 10760239
- PMCID: PMC139848
- DOI: 10.1105/tpc.12.4.493
Structural requirements for ligand binding by a probable plant vacuolar sorting receptor
Abstract
How sorting receptors recognize amino acid determinants on polypeptide ligands and respond to pH changes for ligand binding or release is unknown. The plant vacuolar sorting receptor BP-80 binds polypeptide ligands with a central Asn-Pro-Ile-Arg (NPIR) motif. tBP-80, a soluble form of the receptor lacking transmembrane and cytoplasmic sequences, binds the peptide SSSFADSNPIRPVTDRAASTYC as a monomer with a specificity indistinguishable from that of BP-80. tBP-80 contains an N-terminal region homologous to ReMembR-H2 (RMR) protein lumenal domains, a unique central region, and three C-terminal epidermal growth factor (EGF) repeats. By protease digestion of purified secreted tBP-80, and from ligand binding studies with a secreted protein lacking the EGF repeats, we defined three protease-resistant structural domains: an N-terminal/RMR homology domain connected to a central domain, which together determine the NPIR-specific ligand binding site, and a C-terminal EGF repeat domain that alters the conformation of the other two domains to enhance ligand binding. A fragment representing the central domain plus the C-terminal domain could bind ligand but was not specific for NPIR. These results indicate that two tBP-80 binding sites recognize two separate ligand determinants: a non-NPIR site defined by the central domain-EGF repeat domain structure and an NPIR-specific site contributed by the interaction of the N-terminal/RMR homology domain and the central domain.
Figures
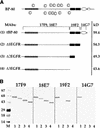
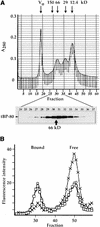
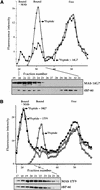
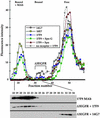
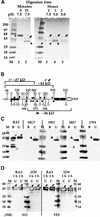
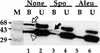
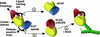
Similar articles
-
Molecular cloning and further characterization of a probable plant vacuolar sorting receptor.Plant Physiol. 1997 Sep;115(1):29-39. doi: 10.1104/pp.115.1.29. Plant Physiol. 1997. PMID: 9306690 Free PMC article.
-
Structure and function of procollagen C-proteinase (mTolloid) domains determined by protease digestion, circular dichroism, binding to procollagen type I, and computer modeling.Biochemistry. 2000 Mar 28;39(12):3231-9. doi: 10.1021/bi992312o. Biochemistry. 2000. PMID: 10727214
-
Molecular basis of ligand recognition by integrin alpha 5beta 1. I. Specificity of ligand binding is determined by amino acid sequences in the second and third NH2-terminal repeats of the alpha subunit.J Biol Chem. 2000 Jul 7;275(27):20324-36. doi: 10.1074/jbc.M000572200. J Biol Chem. 2000. PMID: 10764748
-
Plant RMR proteins: unique vacuolar sorting receptors that couple ligand sorting with membrane internalization.FEBS J. 2011 Jan;278(1):59-68. doi: 10.1111/j.1742-4658.2010.07923.x. Epub 2010 Nov 16. FEBS J. 2011. PMID: 21078125 Review.
-
Molecular characteristics of insect vitellogenins and vitellogenin receptors.Insect Biochem Mol Biol. 1998 May-Jun;28(5-6):277-300. doi: 10.1016/s0965-1748(97)00110-0. Insect Biochem Mol Biol. 1998. PMID: 9692232 Review.
Cited by
-
The internal propeptide of the ricin precursor carries a sequence-specific determinant for vacuolar sorting.Plant Physiol. 2001 May;126(1):167-75. doi: 10.1104/pp.126.1.167. Plant Physiol. 2001. PMID: 11351080 Free PMC article.
-
Functional implication of the homotrimeric multidomain vacuolar sorting receptor 1 (VSR1) from Arabidopsis thaliana.Sci Rep. 2024 Apr 26;14(1):9622. doi: 10.1038/s41598-024-57975-2. Sci Rep. 2024. PMID: 38671060 Free PMC article.
-
Vacuolar Sorting Receptor-Mediated Trafficking of Soluble Vacuolar Proteins in Plant Cells.Plants (Basel). 2014 Aug 25;3(3):392-408. doi: 10.3390/plants3030392. Plants (Basel). 2014. PMID: 27135510 Free PMC article. Review.
-
Toward understanding vesicle traffic and the guard cell model.New Phytol. 2002 Mar;153(3):405-413. doi: 10.1046/j.0028-646X.2001.00341.x. Epub 2002 Mar 5. New Phytol. 2002. PMID: 33863212 Review.
-
Tissue-specific and developmentally regulated expression of a cluster of tandemly arrayed cell wall-associated kinase-like kinase genes in Arabidopsis.Plant Physiol. 2003 Dec;133(4):1732-46. doi: 10.1104/pp.103.028530. Epub 2003 Oct 23. Plant Physiol. 2003. PMID: 14576286 Free PMC article.
References
-
- Davis, C.G. (1990). The many faces of epidermal growth factor repeats. New Biol. 2, 410–419. - PubMed
Publication types
MeSH terms
Substances
LinkOut - more resources
Full Text Sources