GATE-16, a membrane transport modulator, interacts with NSF and the Golgi v-SNARE GOS-28
- PMID: 10747018
- PMCID: PMC310219
- DOI: 10.1093/emboj/19.7.1494
GATE-16, a membrane transport modulator, interacts with NSF and the Golgi v-SNARE GOS-28
Abstract
Membrane proteins located on vesicles (v-SNAREs) and on the target membrane (t-SNAREs) mediate specific recognition and, possibly, fusion between a transport vesicle and its target membrane. The activity of SNARE molecules is regulated by several soluble cytosolic proteins. We have cloned a bovine brain cDNA encoding a conserved 117 amino acid polypeptide, denoted Golgi-associated ATPase Enhancer of 16 kDa (GATE-16), that functions as a soluble transport factor. GATE-16 interacts with N-ethylmaleimidesensitive factor (NSF) and significantly stimulates its ATPase activity. It also interacts with the Golgi v-SNARE GOS-28 in an NSF-dependent manner. We propose that GATE-16 modulates intra-Golgi transport through coupling between NSF activity and SNAREs activation.
Figures
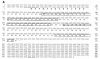
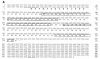
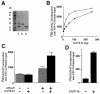
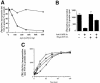
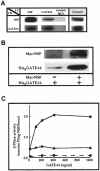
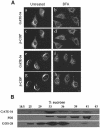
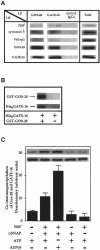
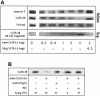
Similar articles
-
Sequential SNARE disassembly and GATE-16-GOS-28 complex assembly mediated by distinct NSF activities drives Golgi membrane fusion.J Cell Biol. 2002 Jun 24;157(7):1161-73. doi: 10.1083/jcb.200202082. Epub 2002 Jun 17. J Cell Biol. 2002. PMID: 12070132 Free PMC article.
-
An NSF function distinct from ATPase-dependent SNARE disassembly is essential for Golgi membrane fusion.Nat Cell Biol. 1999 Oct;1(6):335-40. doi: 10.1038/14025. Nat Cell Biol. 1999. PMID: 10559959
-
Crystal structure of the amino-terminal domain of N-ethylmaleimide-sensitive fusion protein.Nat Cell Biol. 1999 Jul;1(3):175-82. doi: 10.1038/11097. Nat Cell Biol. 1999. PMID: 10559905
-
Protein interactions implicated in neurotransmitter release.J Physiol Paris. 1998 Apr;92(2):129-33. doi: 10.1016/S0928-4257(98)80150-2. J Physiol Paris. 1998. PMID: 9782456 Review.
-
Fusion of membranes during the acrosome reaction: a tale of two SNAREs.Mol Reprod Dev. 2000 Dec;57(4):309-10. doi: 10.1002/1098-2795(200012)57:4<309::AID-MRD1>3.0.CO;2-W. Mol Reprod Dev. 2000. PMID: 11066058 Review.
Cited by
-
The functional and pathologic relevance of autophagy proteases.J Clin Invest. 2015 Jan;125(1):33-41. doi: 10.1172/JCI73940. Epub 2015 Jan 2. J Clin Invest. 2015. PMID: 25654548 Free PMC article. Review.
-
AIM/LIR-based fluorescent sensors-new tools to monitor mAtg8 functions.Autophagy. 2018;14(6):1074-1078. doi: 10.1080/15548627.2018.1454238. Epub 2018 May 11. Autophagy. 2018. PMID: 29749795 Free PMC article.
-
Genetics of GABAergic signaling in nicotine and alcohol dependence.Hum Genet. 2012 Jun;131(6):843-55. doi: 10.1007/s00439-011-1108-4. Epub 2011 Nov 3. Hum Genet. 2012. PMID: 22048727 Free PMC article. Review.
-
Atg8ylation as a general membrane stress and remodeling response.Cell Stress. 2021 Aug 12;5(9):128-142. doi: 10.15698/cst2021.09.255. eCollection 2021 Sep. Cell Stress. 2021. PMID: 34527862 Free PMC article. Review.
-
GABARAPL1 tumor suppressive function is independent of its conjugation to autophagosomes in MCF-7 breast cancer cells.Oncotarget. 2017 Jul 27;8(34):55998-56020. doi: 10.18632/oncotarget.19639. eCollection 2017 Aug 22. Oncotarget. 2017. PMID: 28915569 Free PMC article.
References
-
- Balch W.E., Dunphy, W.G., Braell, W.A. and Rothman, J.E. (1984) Reconstitution of the transport of protein between successive compartments of the Golgi measured by the coupled incorporation of N-acetylglucosamine. Cell, 39, 405–416. - PubMed
-
- Clary D.O., Griff, I.C. and Rothman, J.E. (1990) SNAPs, a family of NSF attachment proteins involved in intracellular membrane fusion in animals and yeast. Cell, 61, 709–721. - PubMed
Publication types
MeSH terms
Substances
Associated data
- Actions
LinkOut - more resources
Full Text Sources
Molecular Biology Databases