Keratocytes generate traction forces in two phases
- PMID: 10564269
- PMCID: PMC25676
- DOI: 10.1091/mbc.10.11.3745
Keratocytes generate traction forces in two phases
Abstract
Forces generated by goldfish keratocytes and Swiss 3T3 fibroblasts have been measured with nanonewton precision and submicrometer spatial resolution. Differential interference contrast microscopy was used to visualize deformations produced by traction forces in elastic substrata, and interference reflection microscopy revealed sites of cell-substratum adhesions. Force ranged from a few nanonewtons at submicrometer spots under the lamellipodium to several hundred nanonewtons under the cell body. As cells moved forward, centripetal forces were applied by lamellipodia at sites that remained stationary on the substratum. Force increased and abruptly became lateral at the boundary of the lamellipodium and the cell body. When the cell retracted at its posterior margin, cell-substratum contact area decreased more rapidly than force, so that stress (force divided by area) increased as the cell pulled away. An increase in lateral force was associated with widening of the cell body. These mechanical data suggest an integrated, two-phase mechanism of cell motility: (1) low forces in the lamellipodium are applied in the direction of cortical flow and cause the cell body to be pulled forward; and (2) a component of force at the flanks pulls the rear margins forward toward the advancing cell body, whereas a large lateral component contributes to detachment of adhesions without greatly perturbing forward movement.
Figures
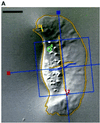
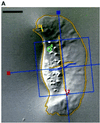
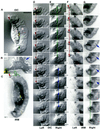
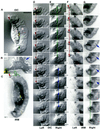
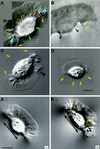
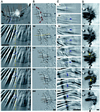
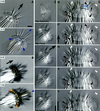
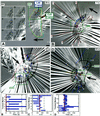
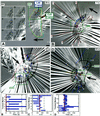
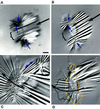
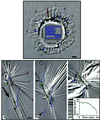
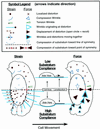
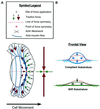
Similar articles
-
Keratocytes pull with similar forces on their dorsal and ventral surfaces.J Cell Biol. 1999 Dec 13;147(6):1313-24. doi: 10.1083/jcb.147.6.1313. J Cell Biol. 1999. PMID: 10601343 Free PMC article.
-
Force transmission in migrating cells.J Cell Biol. 2010 Jan 25;188(2):287-97. doi: 10.1083/jcb.200906139. J Cell Biol. 2010. PMID: 20100912 Free PMC article.
-
Locomotion of fish epidermal keratocytes on spatially selective adhesion patterns.Cell Motil Cytoskeleton. 2007 Nov;64(11):856-67. doi: 10.1002/cm.20230. Cell Motil Cytoskeleton. 2007. PMID: 17712861
-
Forces in cell locomotion.Biochem Soc Symp. 1999;65:299-314. Biochem Soc Symp. 1999. PMID: 10320946 Review.
-
On the mechanisms of cortical actin flow and its role in cytoskeletal organisation of fibroblasts.Symp Soc Exp Biol. 1993;47:35-56. Symp Soc Exp Biol. 1993. PMID: 8165576 Review.
Cited by
-
Stretchy proteins on stretchy substrates: the important elements of integrin-mediated rigidity sensing.Dev Cell. 2010 Aug 17;19(2):194-206. doi: 10.1016/j.devcel.2010.07.018. Dev Cell. 2010. PMID: 20708583 Free PMC article. Review.
-
Staurosporine induces lamellipodial widening in locomoting fish keratocytes by abolishing the gradient from radial extension of leading edge.Biophysics (Nagoya-shi). 2011 Sep 27;7:69-75. doi: 10.2142/biophysics.7.69. eCollection 2011. Biophysics (Nagoya-shi). 2011. PMID: 27857594 Free PMC article.
-
Collective epithelial cell sheet adhesion and migration on polyelectrolyte multilayers with uniform and gradients of compliance.Exp Cell Res. 2016 Aug 1;346(1):17-29. doi: 10.1016/j.yexcr.2016.06.002. Epub 2016 Jun 9. Exp Cell Res. 2016. PMID: 27292313 Free PMC article.
-
Traction forces mediated by alpha6beta4 integrin: implications for basement membrane organization and tumor invasion.Mol Biol Cell. 2001 Dec;12(12):4030-43. doi: 10.1091/mbc.12.12.4030. Mol Biol Cell. 2001. PMID: 11739798 Free PMC article.
-
Chaperonin containing T-complex polypeptide subunit eta (CCT-eta) is a specific regulator of fibroblast motility and contractility.PLoS One. 2010 Apr 30;5(4):e10063. doi: 10.1371/journal.pone.0010063. PLoS One. 2010. PMID: 20442790 Free PMC article.
References
-
- Abercrombie M, Heaysman JEM, Pegrum SM. The locomotion of fibroblasts in culture. I. Movements of the leading edge. Exp Cell Res. 1970;59:393–398. - PubMed
-
- Bailey B, Farkas DL, Taylor DL, Lanni F. Enhancement of axial resolution in fluorescence microscopy by standing-wave excitation. Nature. 1993;366:44–48. - PubMed
-
- Bereiter-Hahn J, Luers H. The role of elasticity in the motile behavior of cells. In: Akkas N, editor. Biomechanics of Active Movement and Division of Cells, NATO ASI Series. H84. Berlin: Springer-Verlag; 1994. pp. 181–230.
Publication types
MeSH terms
Substances
Grants and funding
LinkOut - more resources
Full Text Sources
Other Literature Sources
Research Materials