A cytoplasmic dynein heavy chain is required for oscillatory nuclear movement of meiotic prophase and efficient meiotic recombination in fission yeast
- PMID: 10366596
- PMCID: PMC2133150
- DOI: 10.1083/jcb.145.6.1233
A cytoplasmic dynein heavy chain is required for oscillatory nuclear movement of meiotic prophase and efficient meiotic recombination in fission yeast
Abstract
Meiotic recombination requires pairing of homologous chromosomes, the mechanisms of which remain largely unknown. When pairing occurs during meiotic prophase in fission yeast, the nucleus oscillates between the cell poles driven by astral microtubules. During these oscillations, the telomeres are clustered at the spindle pole body (SPB), located at the leading edge of the moving nucleus and the rest of each chromosome dangles behind. Here, we show that the oscillatory nuclear movement of meiotic prophase is dependent on cytoplasmic dynein. We have cloned the gene encoding a cytoplasmic dynein heavy chain of fission yeast. Most of the cells disrupted for the gene show no gross defect during mitosis and complete meiosis to form four viable spores, but they lack the nuclear movements of meiotic prophase. Thus, the dynein heavy chain is required for these oscillatory movements. Consistent with its essential role in such nuclear movement, dynein heavy chain tagged with green fluorescent protein (GFP) is localized at astral microtubules and the SPB during the movements. In dynein-disrupted cells, meiotic recombination is significantly reduced, indicating that the dynein function is also required for efficient meiotic recombination. In accordance with the reduced recombination, which leads to reduced crossing over, chromosome missegregation is increased in the mutant. Moreover, both the formation of a single cluster of centromeres and the colocalization of homologous regions on a pair of homologous chromosomes are significantly inhibited in the mutant. These results strongly suggest that the dynein-driven nuclear movements of meiotic prophase are necessary for efficient pairing of homologous chromosomes in fission yeast, which in turn promotes efficient meiotic recombination.
Figures
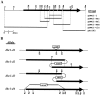
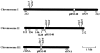
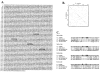
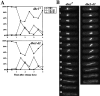

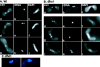
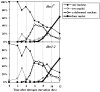
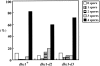
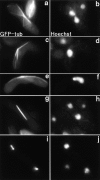
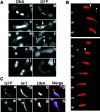
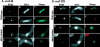
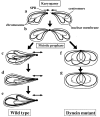
Similar articles
-
Contribution of dynein light intermediate and intermediate chains to subcellular localization of the dynein-dynactin motor complex in Schizosaccharomyces pombe.Genes Cells. 2010 Apr 1;15(4):359-72. doi: 10.1111/j.1365-2443.2010.01386.x. Epub 2010 Mar 14. Genes Cells. 2010. PMID: 20298435
-
The 14-kDa dynein light chain-family protein Dlc1 is required for regular oscillatory nuclear movement and efficient recombination during meiotic prophase in fission yeast.Mol Biol Cell. 2002 Mar;13(3):930-46. doi: 10.1091/mbc.01-11-0543. Mol Biol Cell. 2002. PMID: 11907273 Free PMC article.
-
Mcp5, a meiotic cell cortex protein, is required for nuclear movement mediated by dynein and microtubules in fission yeast.J Cell Biol. 2006 Apr 10;173(1):27-33. doi: 10.1083/jcb.200512129. Epub 2006 Apr 3. J Cell Biol. 2006. PMID: 16585273 Free PMC article.
-
How do meiotic chromosomes meet their homologous partners?: lessons from fission yeast.Bioessays. 2001 Jun;23(6):526-33. doi: 10.1002/bies.1072. Bioessays. 2001. PMID: 11385632 Review.
-
Homologous chromosome pairing in Schizosaccharomyces pombe.Yeast. 2006 Oct 15;23(13):977-89. doi: 10.1002/yea.1403. Yeast. 2006. PMID: 17072890 Review.
Cited by
-
Microtubule minus end motors kinesin-14 and dynein drive nuclear congression in parallel pathways.J Cell Biol. 2015 Apr 13;209(1):47-58. doi: 10.1083/jcb.201409087. J Cell Biol. 2015. PMID: 25869666 Free PMC article.
-
Global roles of Ste11p, cell type, and pheromone in the control of gene expression during early sexual differentiation in fission yeast.Proc Natl Acad Sci U S A. 2006 Oct 17;103(42):15517-22. doi: 10.1073/pnas.0603403103. Epub 2006 Oct 9. Proc Natl Acad Sci U S A. 2006. PMID: 17032641 Free PMC article.
-
Nuclear Mechanics in the Fission Yeast.Cells. 2019 Oct 20;8(10):1285. doi: 10.3390/cells8101285. Cells. 2019. PMID: 31635174 Free PMC article. Review.
-
Meiotic telomere protein Ndj1p is required for meiosis-specific telomere distribution, bouquet formation and efficient homologue pairing.J Cell Biol. 2000 Oct 2;151(1):95-106. doi: 10.1083/jcb.151.1.95. J Cell Biol. 2000. PMID: 11018056 Free PMC article.
-
pkl1(+)and klp2(+): Two kinesins of the Kar3 subfamily in fission yeast perform different functions in both mitosis and meiosis.Mol Biol Cell. 2001 Nov;12(11):3476-88. doi: 10.1091/mbc.12.11.3476. Mol Biol Cell. 2001. PMID: 11694582 Free PMC article.
References
Publication types
MeSH terms
Substances
Associated data
- Actions
Grants and funding
LinkOut - more resources
Full Text Sources
Molecular Biology Databases