Mechanism of regulation of hsp70 chaperones by DnaJ cochaperones
- PMID: 10318904
- PMCID: PMC21880
- DOI: 10.1073/pnas.96.10.5452
Mechanism of regulation of hsp70 chaperones by DnaJ cochaperones
Abstract
Hsp70 chaperones assist a large variety of protein folding processes within the entire lifespan of proteins. Central to these activities is the regulation of Hsp70 by DnaJ cochaperones. DnaJ stimulates Hsp70 to hydrolyze ATP, a key step that closes its substrate-binding cavity and thus allows stable binding of substrate. We show that DnaJ stimulates ATP hydrolysis by Escherichia coli Hsp70, DnaK, very efficiently to >1000-fold, but only if present at high (micromolar) concentration. In contrast, the chaperone activity of DnaK in luciferase refolding was maximal at several hundredfold lower concentration of DnaJ. However, DnaJ was capable of maximally stimulating the DnaK ATPase even at this low concentration, provided that protein substrate was present, indicating synergistic action of DnaJ and substrate. Peptide substrates were poorly effective in this synergistic action. DnaJ action required binding of protein substrates to the central hydrophobic pocket of the substrate-binding cavity of DnaK, as evidenced by the reduced ability of DnaJ to stimulate ATP hydrolysis by a DnaK mutant with defects in substrate binding. At high concentrations, DnaJ itself served as substrate for DnaK in a process considered to be unphysiological. Mutant analysis furthermore revealed that DnaJ-mediated stimulation of ATP hydrolysis requires communication between the ATPase and substrate-binding domains of DnaK. This mechanism thus allows DnaJ to tightly couple ATP hydrolysis by DnaK with substrate binding and to avoid jamming of the DnaK chaperone with peptides. It probably is conserved among Hsp70 family members and is proposed to account for their functional diversity.
Figures
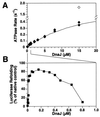
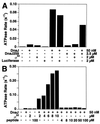
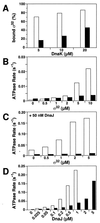
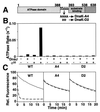
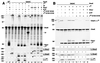
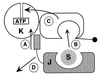
Similar articles
-
Investigation of the interaction between DnaK and DnaJ by surface plasmon resonance spectroscopy.J Mol Biol. 1999 Jun 18;289(4):1131-44. doi: 10.1006/jmbi.1999.2844. J Mol Biol. 1999. PMID: 10369787
-
Mutations in the DnaK chaperone affecting interaction with the DnaJ cochaperone.Proc Natl Acad Sci U S A. 1998 Dec 22;95(26):15229-34. doi: 10.1073/pnas.95.26.15229. Proc Natl Acad Sci U S A. 1998. PMID: 9860951 Free PMC article.
-
The ATP hydrolysis-dependent reaction cycle of the Escherichia coli Hsp70 system DnaK, DnaJ, and GrpE.Proc Natl Acad Sci U S A. 1994 Oct 25;91(22):10345-9. doi: 10.1073/pnas.91.22.10345. Proc Natl Acad Sci U S A. 1994. PMID: 7937953 Free PMC article.
-
Mechanism of the targeting action of DnaJ in the DnaK molecular chaperone system.J Biol Chem. 2003 May 23;278(21):19038-43. doi: 10.1074/jbc.M300756200. Epub 2003 Mar 24. J Biol Chem. 2003. PMID: 12654915
-
Interferon-gamma is a target for binding and folding by both Escherichia coli chaperone model systems GroEL/GroES and DnaK/DnaJ/GrpE.Biochimie. 1998 Aug-Sep;80(8-9):729-37. doi: 10.1016/s0300-9084(99)80026-1. Biochimie. 1998. PMID: 9865495 Review.
Cited by
-
The HSP70 family and cancer.Carcinogenesis. 2013 Jun;34(6):1181-8. doi: 10.1093/carcin/bgt111. Epub 2013 Apr 4. Carcinogenesis. 2013. PMID: 23563090 Free PMC article. Review.
-
Mutation of GGMP Repeat Segments of Plasmodium falciparum Hsp70-1 Compromises Chaperone Function and Hop Co-Chaperone Binding.Int J Mol Sci. 2021 Feb 23;22(4):2226. doi: 10.3390/ijms22042226. Int J Mol Sci. 2021. PMID: 33672387 Free PMC article.
-
Combining crystallography and EPR: crystal and solution structures of the multidomain cochaperone DnaJ.Acta Crystallogr D Biol Crystallogr. 2013 Aug;69(Pt 8):1540-52. doi: 10.1107/S0907444913010640. Epub 2013 Jul 19. Acta Crystallogr D Biol Crystallogr. 2013. PMID: 23897477 Free PMC article.
-
An interdomain sector mediating allostery in Hsp70 molecular chaperones.Mol Syst Biol. 2010 Sep 21;6:414. doi: 10.1038/msb.2010.65. Mol Syst Biol. 2010. PMID: 20865007 Free PMC article.
-
Ischemic preconditioning prevents protein aggregation after transient cerebral ischemia.Neuroscience. 2005;134(1):69-80. doi: 10.1016/j.neuroscience.2005.03.036. Neuroscience. 2005. PMID: 15939539 Free PMC article.
References
-
- Bukau B, editor. Molecular Chaperones and Folding Catalysts—Regulation, Cellular Function and Mechanisms. Amsterdam: Harwood; 1999.
-
- Bukau B, Horwich A L. Cell. 1998;92:351–366. - PubMed
-
- Hartl F U. Nature (London) 1996;381:571–580. - PubMed
-
- Gething M-J. Nature (London) 1997;388:329–331. - PubMed
-
- Schmid D, Baici A, Gehring H, Christen P. Science. 1994;263:971–973. - PubMed
Publication types
MeSH terms
Substances
LinkOut - more resources
Full Text Sources
Other Literature Sources