Oscillatory coupling of hippocampal pyramidal cells and interneurons in the behaving Rat
- PMID: 9870957
- PMCID: PMC6782375
- DOI: 10.1523/JNEUROSCI.19-01-00274.1999
Oscillatory coupling of hippocampal pyramidal cells and interneurons in the behaving Rat
Abstract
We examined whether excitation and inhibition are balanced in hippocampal cortical networks. Extracellular field and single-unit activity were recorded by multiple tetrodes and multisite silicon probes to reveal the timing of the activity of hippocampal CA1 pyramidal cells and classes of interneurons during theta waves and sharp wave burst (SPW)-associated field ripples. The somatic and dendritic inhibition of pyramidal cells was deduced from the activity of interneurons in the pyramidal layer [int(p)] and in the alveus and st. oriens [int(a/o)], respectively. Int(p) and int(a/o) discharged an average of 60 and 20 degrees before the population discharge of pyramidal cells during the theta cycle, respectively. SPW ripples were associated with a 2.5-fold net increase of excitation. The discharge frequency of int(a/o) increased, decreased ("anti-SPW" cells), or did not change ("SPW-independent" cells) during SPW, suggesting that not all interneurons are innervated by pyramidal cells. Int(p) either fired together with (unimodal cells) or both before and after (bimodal cells) the pyramidal cell burst. During fast-ripple oscillation, the activity of interneurons in both the int(p) and int(a/o) groups lagged the maximum discharge probability of pyramidal neurons by 1-2 msec. Network state changes, as reflected by field activity, covaried with changes in the spike train dynamics of single cells and their interactions. Summed activity of parallel-recorded interneurons, but not of pyramidal cells, reliably predicted theta cycles, whereas the reverse was true for the ripple cycles of SPWs. We suggest that network-driven excitability changes provide temporal windows of opportunity for single pyramidal cells to suppress, enable, or facilitate selective synaptic inputs.
Figures
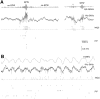
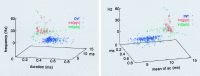
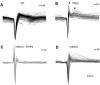
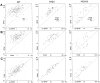
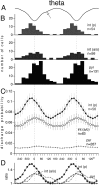
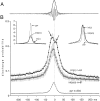
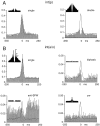
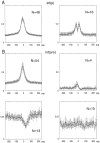
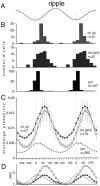
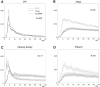
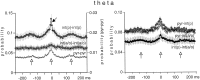
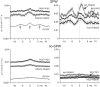
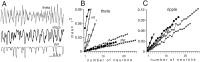
Similar articles
-
Fast network oscillations in the hippocampal CA1 region of the behaving rat.J Neurosci. 1999 Aug 15;19(16):RC20. doi: 10.1523/JNEUROSCI.19-16-j0001.1999. J Neurosci. 1999. PMID: 10436076 Free PMC article.
-
Interactions between hippocampus and medial septum during sharp waves and theta oscillation in the behaving rat.J Neurosci. 1999 Jul 15;19(14):6191-9. doi: 10.1523/JNEUROSCI.19-14-06191.1999. J Neurosci. 1999. PMID: 10407055 Free PMC article.
-
Sharp wave-associated high-frequency oscillation (200 Hz) in the intact hippocampus: network and intracellular mechanisms.J Neurosci. 1995 Jan;15(1 Pt 1):30-46. doi: 10.1523/JNEUROSCI.15-01-00030.1995. J Neurosci. 1995. PMID: 7823136 Free PMC article.
-
Cellular bases of hippocampal EEG in the behaving rat.Brain Res. 1983 Oct;287(2):139-71. doi: 10.1016/0165-0173(83)90037-1. Brain Res. 1983. PMID: 6357356 Review.
-
[Hippocampal ripple oscillations (200 Hz) in mechanisms of memory consolidation].Usp Fiziol Nauk. 2002 Oct-Dec;33(4):34-42. Usp Fiziol Nauk. 2002. PMID: 12449805 Review. Russian.
Cited by
-
GABAergic Interneurons are Required for Generation of Slow CA1 Oscillation in Rat Hippocampus.Neurosci Bull. 2016 Aug;32(4):363-73. doi: 10.1007/s12264-016-0049-2. Epub 2016 Jul 20. Neurosci Bull. 2016. PMID: 27439706 Free PMC article.
-
Excitatory Inputs Determine Phase-Locking Strength and Spike-Timing of CA1 Stratum Oriens/Alveus Parvalbumin and Somatostatin Interneurons during Intrinsically Generated Hippocampal Theta Rhythm.J Neurosci. 2016 Jun 22;36(25):6605-22. doi: 10.1523/JNEUROSCI.3951-13.2016. J Neurosci. 2016. PMID: 27335395 Free PMC article.
-
A model for attentional information routing through coherence predicts biased competition and multistable perception.J Neurophysiol. 2015 Sep;114(3):1593-605. doi: 10.1152/jn.01038.2014. Epub 2015 Jun 24. J Neurophysiol. 2015. PMID: 26108958 Free PMC article.
-
Cell-type-specific recruitment of amygdala interneurons to hippocampal theta rhythm and noxious stimuli in vivo.Neuron. 2012 Jun 21;74(6):1059-74. doi: 10.1016/j.neuron.2012.04.022. Neuron. 2012. PMID: 22726836 Free PMC article.
-
Spread of pathological human Tau from neurons to oligodendrocytes and loss of high-firing pyramidal neurons in aging mice.Cell Rep. 2022 Nov 15;41(7):111646. doi: 10.1016/j.celrep.2022.111646. Cell Rep. 2022. PMID: 36384116 Free PMC article.
References
-
- Abeles M. Local cortical circuits: an electrophysiological study. Springer; Berlin: 1982.
-
- Abeles M, Goldstein MH. Multispike train analysis. Proc IEEE. 1977;65:762–773.
-
- Bair W, Koch C. Temporal precision of spike trains in extrastriate cortex of the behaving macaque monkey. Neural Comput. 1996;8:1185–1202. - PubMed
-
- Bankman IN, Johnson KO, Schneide W. Optimal detection, classification, and superposition resolution in neural waveform recordings. IEEE Trans Biomed Eng. 1993;40:836–841. - PubMed
Publication types
MeSH terms
Grants and funding
LinkOut - more resources
Full Text Sources
Other Literature Sources
Miscellaneous