Paralemmin, a prenyl-palmitoyl-anchored phosphoprotein abundant in neurons and implicated in plasma membrane dynamics and cell process formation
- PMID: 9813098
- PMCID: PMC2148134
- DOI: 10.1083/jcb.143.3.795
Paralemmin, a prenyl-palmitoyl-anchored phosphoprotein abundant in neurons and implicated in plasma membrane dynamics and cell process formation
Abstract
We report the identification and initial characterization of paralemmin, a putative new morphoregulatory protein associated with the plasma membrane. Paralemmin is highly expressed in the brain but also less abundantly in many other tissues and cell types. cDNAs from chicken, human, and mouse predict acidic proteins of 42 kD that display a pattern of sequence cassettes with high inter-species conservation separated by poorly conserved linker sequences. Prenylation and palmitoylation of a COOH-terminal cluster of three cysteine residues confers hydrophobicity and membrane association to paralemmin. Paralemmin is also phosphorylated, and its mRNA is differentially spliced in a tissue-specific and developmentally regulated manner. Differential splicing, lipidation, and phosphorylation contribute to electrophoretic heterogeneity that results in an array of multiple bands on Western blots, most notably in brain. Paralemmin is associated with the cytoplasmic face of the plasma membranes of postsynaptic specializations, axonal and dendritic processes and perikarya, and also appears to be associated with an intracellular vesicle pool. It does not line the neuronal plasmalemma continuously but in clusters and patches. Its molecular and morphological properties are reminiscent of GAP-43, CAP-23, and MARCKS, proteins implicated in plasma membrane dynamics. Overexpression in several cell lines shows that paralemmin concentrates at sites of plasma membrane activity such as filopodia and microspikes, and induces cell expansion and process formation. The lipidation motif is essential for this morphogenic activity. We propose a function for paralemmin in the control of cell shape, e.g., through an involvement in membrane flow or in membrane-cytoskeleton interaction.
Figures
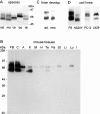
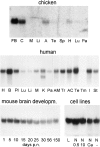
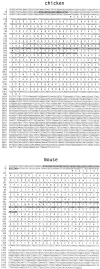
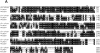
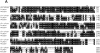
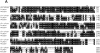
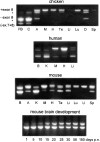
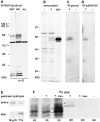
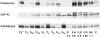
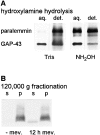
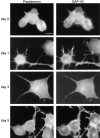
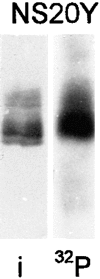
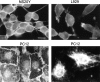
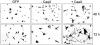
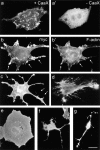
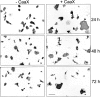
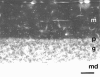
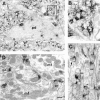
Similar articles
-
The paralemmin protein family: identification of paralemmin-2, an isoform differentially spliced to AKAP2/AKAP-KL, and of palmdelphin, a more distant cytosolic relative.Biochem Biophys Res Commun. 2001 Aug 3;285(5):1369-76. doi: 10.1006/bbrc.2001.5329. Biochem Biophys Res Commun. 2001. PMID: 11478809
-
Cellular and subcellular localization of paralemmin-1, a protein involved in cell shape control, in the rat brain, adrenal gland and kidney.Histochem Cell Biol. 2007 Jan;127(1):13-30. doi: 10.1007/s00418-006-0209-y. Epub 2006 Jul 18. Histochem Cell Biol. 2007. PMID: 16847661
-
Molecular characterization and immunohistochemical localization of palmdelphin, a cytosolic isoform of the paralemmin protein family implicated in membrane dynamics.Eur J Cell Biol. 2005 Nov;84(11):853-66. doi: 10.1016/j.ejcb.2005.07.002. Eur J Cell Biol. 2005. PMID: 16323283
-
Paralemmin-1, a modulator of filopodia induction is required for spine maturation.Mol Biol Cell. 2008 May;19(5):2026-38. doi: 10.1091/mbc.e07-08-0802. Epub 2008 Feb 20. Mol Biol Cell. 2008. PMID: 18287537 Free PMC article.
-
Neuronal cell membranes and brain aging.Prog Brain Res. 1986;70:239-54. doi: 10.1016/s0079-6123(08)64308-3. Prog Brain Res. 1986. PMID: 3033742 Review. No abstract available.
Cited by
-
Palmdelphin Deficiency Evokes NF-κB Signaling in Valvular Endothelial Cells and Aggravates Aortic Valvular Remodeling.JACC Basic Transl Sci. 2023 Aug 30;8(11):1457-1472. doi: 10.1016/j.jacbts.2023.06.004. eCollection 2023 Nov. JACC Basic Transl Sci. 2023. PMID: 38093741 Free PMC article.
-
Neurobeachin: A protein kinase A-anchoring, beige/Chediak-higashi protein homolog implicated in neuronal membrane traffic.J Neurosci. 2000 Dec 1;20(23):8551-65. doi: 10.1523/JNEUROSCI.20-23-08551.2000. J Neurosci. 2000. PMID: 11102458 Free PMC article.
-
Receptor-like Molecules on Human Intestinal Epithelial Cells Interact with an Adhesion Factor from Lactobacillus reuteri.Biosci Microbiota Food Health. 2012;31(4):93-102. doi: 10.12938/bmfh.31.93. Epub 2012 Oct 25. Biosci Microbiota Food Health. 2012. PMID: 24936355 Free PMC article.
-
Regulation of dendritic branching and filopodia formation in hippocampal neurons by specific acylated protein motifs.Mol Biol Cell. 2004 May;15(5):2205-17. doi: 10.1091/mbc.e03-07-0493. Epub 2004 Feb 20. Mol Biol Cell. 2004. PMID: 14978216 Free PMC article.
-
Role of a Pdlim5:PalmD complex in directing dendrite morphology.Front Cell Neurosci. 2024 Feb 13;18:1315941. doi: 10.3389/fncel.2024.1315941. eCollection 2024. Front Cell Neurosci. 2024. PMID: 38414752 Free PMC article.
References
-
- Adamson P, Marshall CJ, Hall A, Tilbrook PA. Post-translational modifications of p21rho proteins. J Biol Chem. 1992;267:20033–20038. - PubMed
-
- Aigner L, Arber S, Kapfhammer JP, Laux T, Schneider C, Botteri F, Brenner H-R, Caroni P. Overexpression of the neural growth-associated protein GAP-43 induces nerve sprouting in the adult nervous system of transgenic mice. Cell. 1995;83:269–278. - PubMed
-
- Babitch JA, Breithaupt TB, Chiu T-C, Garadi R, Helseth DL. Preparation of chick brain synaptosomes and synaptosomal membranes. Biochim Biophys Acta. 1976;433:75–89. - PubMed
Publication types
MeSH terms
Substances
Associated data
- Actions
- Actions
- Actions
LinkOut - more resources
Full Text Sources
Molecular Biology Databases
Miscellaneous