The dynamin-related GTPase, Dnm1p, controls mitochondrial morphology in yeast
- PMID: 9786946
- PMCID: PMC2132834
- DOI: 10.1083/jcb.143.2.333
The dynamin-related GTPase, Dnm1p, controls mitochondrial morphology in yeast
Abstract
The Saccharomyces cerevisiae Dnm1 protein is structurally related to dynamin, a GTPase required for membrane scission during endocytosis. Here we show that Dnm1p is essential for the maintenance of mitochondrial morphology. Disruption of the DNM1 gene causes the wild-type network of tubular mitochondrial membranes to collapse to one side of the cell but does not affect the morphology or distribution of other cytoplasmic organelles. Dnm1 proteins containing point mutations in the predicted GTP-binding domain or completely lacking the GTP-binding domain fail to rescue mitochondrial morphology defects in a dnm1 mutant and induce dominant mitochondrial morphology defects in wild-type cells. Indirect immunofluorescence reveals that Dnm1p is distributed in punctate structures at the cell cortex that colocalize with the mitochondrial compartment. These Dnm1p-containing structures remain associated with the spherical mitochondria found in an mdm10 mutant strain. In addition, a portion of Dnm1p cofractionates with mitochondrial membranes during differential sedimentation and sucrose gradient fractionation of wild-type cells. Our results demonstrate that Dnm1p is required for the cortical distribution of the mitochondrial network in yeast, a novel function for a dynamin-related protein.
Figures
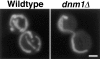
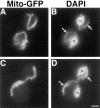
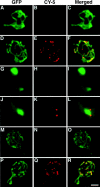
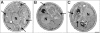
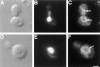
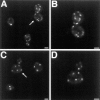
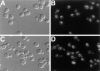
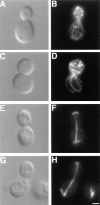
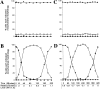
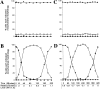
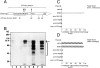
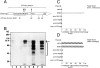
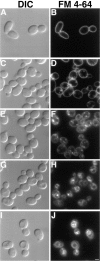
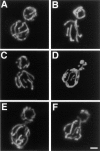
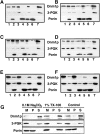
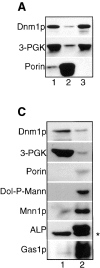
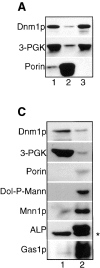
Similar articles
-
DNM1, a dynamin-related gene, participates in endosomal trafficking in yeast.J Cell Biol. 1995 Aug;130(3):553-66. doi: 10.1083/jcb.130.3.553. J Cell Biol. 1995. PMID: 7622557 Free PMC article.
-
The GTPase effector domain sequence of the Dnm1p GTPase regulates self-assembly and controls a rate-limiting step in mitochondrial fission.Mol Biol Cell. 2001 Sep;12(9):2756-66. doi: 10.1091/mbc.12.9.2756. Mol Biol Cell. 2001. PMID: 11553714 Free PMC article.
-
Division versus fusion: Dnm1p and Fzo1p antagonistically regulate mitochondrial shape.J Cell Biol. 1999 Nov 15;147(4):699-706. doi: 10.1083/jcb.147.4.699. J Cell Biol. 1999. PMID: 10562274 Free PMC article.
-
Molecular Basis of Mitochondrial and Peroxisomal Division Machineries.Int J Mol Sci. 2020 Jul 30;21(15):5452. doi: 10.3390/ijms21155452. Int J Mol Sci. 2020. PMID: 32751702 Free PMC article. Review.
-
Mitochondrial division: molecular machinery and physiological functions.Curr Opin Cell Biol. 2011 Aug;23(4):427-34. doi: 10.1016/j.ceb.2011.04.009. Epub 2011 May 10. Curr Opin Cell Biol. 2011. PMID: 21565481 Free PMC article. Review.
Cited by
-
Mechanisms for countering oxidative stress and damage in retinal pigment epithelium.Int Rev Cell Mol Biol. 2012;298:135-77. doi: 10.1016/B978-0-12-394309-5.00004-3. Int Rev Cell Mol Biol. 2012. PMID: 22878106 Free PMC article. Review.
-
Mitochondrial dynamics.New Phytol. 2003 Dec;160(3):463-478. doi: 10.1046/j.1469-8137.2003.00918.x. Epub 2003 Nov 6. New Phytol. 2003. PMID: 33873653 Review.
-
A novel motif in the yeast mitochondrial dynamin Dnm1 is essential for adaptor binding and membrane recruitment.J Cell Biol. 2012 Nov 12;199(4):613-22. doi: 10.1083/jcb.201207079. J Cell Biol. 2012. PMID: 23148233 Free PMC article.
-
Programmed Cell Death Initiation and Execution in Budding Yeast.Genetics. 2015 Aug;200(4):1003-14. doi: 10.1534/genetics.115.179150. Genetics. 2015. PMID: 26272996 Free PMC article. Review.
-
Members of the Arabidopsis dynamin-like gene family, ADL1, are essential for plant cytokinesis and polarized cell growth.Plant Cell. 2003 Apr;15(4):899-913. doi: 10.1105/tpc.009670. Plant Cell. 2003. PMID: 12671086 Free PMC article.
References
-
- Adari H, Lowy DR, Willumsen BM, Der CJ, McCormick F. Guanosine triphosphatase activating protein (GAP) interacts with the p21 raseffector binding domain. Science. 1988;240:518–521. - PubMed
-
- Bakeeva LE, Chentsov YS, Skulachev VP. Mitochondrial framework (reticulum mitochondriale) in rat diaphragm muscle. Biochim Biophys Acta. 1978;501:349–369. - PubMed
-
- Bereiter-Hahn J. Behavior of mitochondria in the living cell. Int Rev Cytol. 1990;122:1–63. - PubMed
Publication types
MeSH terms
Substances
Grants and funding
LinkOut - more resources
Full Text Sources
Molecular Biology Databases