The activity of a highly promiscuous AP-1 element can be confined to neurons by a tissue-selective repressive element
- PMID: 9651209
- PMCID: PMC6793474
- DOI: 10.1523/JNEUROSCI.18-14-05264.1998
The activity of a highly promiscuous AP-1 element can be confined to neurons by a tissue-selective repressive element
Abstract
Tissue-specific gene transcription can be determined by the use of either positive-acting or negative-acting DNA regulatory elements. We have analyzed a promoter from the growth-associated protein 43 (GAP-43) gene and found that it uses both of these mechanisms to achieve its high degree of neuron-specific activity. Two novel transcription factor binding sites, designated Cx1 and Cx2, drive promoter activity in neurons from developing cerebral cortex but not in several other cell types. The promoter also contains an activator protein 1 (AP-1) site that contributes to activity in neurons. The AP-1 site can drive promoter activity in a wide range of non-neuronal cells that express little or no endogenous GAP-43, but only in the absence of a tissue-specific repressive element located downstream of the GAP-43 TATA box. These findings suggest that the GAP-43 repressive element plays an important role in allowing AP-1 signaling pathways to modulate activity of the GAP-43 gene in neurons, without also causing inappropriate activation by AP-1 transcription factors in other cell types.
Figures
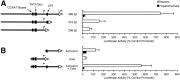
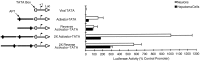
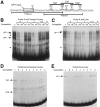
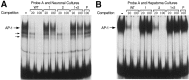
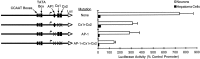
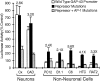
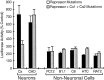
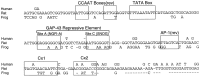
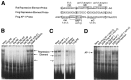
Similar articles
-
Identification of a novel repressive element that contributes to neuron-specific gene expression.J Neurosci. 1997 Oct 15;17(20):7583-93. doi: 10.1523/JNEUROSCI.17-20-07583.1997. J Neurosci. 1997. PMID: 9315881 Free PMC article.
-
Characterization of a nervous system-specific promoter for growth-associated protein 43 gene in Medaka (Oryzias latipes).Brain Res. 2008 Dec 15;1245:1-15. doi: 10.1016/j.brainres.2008.09.071. Epub 2008 Oct 7. Brain Res. 2008. PMID: 18951884
-
Identification of cis-acting regions that contribute to neuron-specific expression of the GAP-43 gene.Biosci Biotechnol Biochem. 2006 Jun;70(6):1492-5. doi: 10.1271/bbb.50576. Biosci Biotechnol Biochem. 2006. PMID: 16794332
-
Binding of cellular repressor protein or the IE2 protein to a cis-acting negative regulatory element upstream of a human cytomegalovirus early promoter.J Virol. 1995 Dec;69(12):7612-21. doi: 10.1128/JVI.69.12.7612-7621.1995. J Virol. 1995. PMID: 7494269 Free PMC article.
-
Cooperation between core binding factor and adjacent promoter elements contributes to the tissue-specific expression of interleukin-3.J Biol Chem. 1996 Jun 14;271(24):14020-7. doi: 10.1074/jbc.271.24.14020. J Biol Chem. 1996. PMID: 8662845
Cited by
-
Multiple pathways transmit neuroprotective effects of gonadal steroids.Endocrine. 2006 Apr;29(2):199-207. doi: 10.1385/ENDO:29:2:199. Endocrine. 2006. PMID: 16785596 Review.
-
Role of transcription factors in peripheral nerve regeneration.Front Mol Neurosci. 2012 Feb 10;5:8. doi: 10.3389/fnmol.2012.00008. eCollection 2012. Front Mol Neurosci. 2012. PMID: 22363260 Free PMC article.
-
Patterns of regulatory activity across diverse human cell types predict tissue identity, transcription factor binding, and long-range interactions.Genome Res. 2013 May;23(5):777-88. doi: 10.1101/gr.152140.112. Epub 2013 Mar 12. Genome Res. 2013. PMID: 23482648 Free PMC article.
-
Transcriptional regulatory regions of gap43 needed in developing and regenerating retinal ganglion cells.Dev Dyn. 2010 Feb;239(2):482-95. doi: 10.1002/dvdy.22190. Dev Dyn. 2010. PMID: 20034105 Free PMC article.
-
ATF3 upregulation in glia during Wallerian degeneration: differential expression in peripheral nerves and CNS white matter.BMC Neurosci. 2004 Mar 4;5:9. doi: 10.1186/1471-2202-5-9. BMC Neurosci. 2004. PMID: 15113454 Free PMC article.
References
-
- Anchan RM, Drake DP, Haines CF, Gerwe EA, LaMantia AS. Disruption of local retinoid-mediated gene expression accompanies abnormal development in the mammalian olfactory pathway. J Comp Neurol. 1997;379:171–184. - PubMed
-
- Bassuk AG, Leiden JM. A direct physical association between ETS and AP-1 transcription factors in normal human T cells. Immunity. 1995;3:223–237. - PubMed
-
- Benda P, Lightbody J, Sato G, Levine L, Sweet W. Differentiated rat glial cell strain in tissue culture. Science. 1968;161:370–371. - PubMed
-
- Brasier AR, Fortin JJ. Nonisotopic assays for reporter gene activity. In: Ausubel FM, Brent R, Kingston RE, Moore DD, Seidman JG, Smith JA, Struhl K, editors. Current protocols in molecular biology. Wiley; New York: 1995. pp. 9.7.12–9.7.14. - PubMed
Publication types
MeSH terms
Substances
Grants and funding
LinkOut - more resources
Full Text Sources
Other Literature Sources
Molecular Biology Databases
Research Materials
Miscellaneous