Role of histone H1 as an architectural determinant of chromatin structure and as a specific repressor of transcription on Xenopus oocyte 5S rRNA genes
- PMID: 9632749
- PMCID: PMC108949
- DOI: 10.1128/MCB.18.7.3668
Role of histone H1 as an architectural determinant of chromatin structure and as a specific repressor of transcription on Xenopus oocyte 5S rRNA genes
Abstract
We explore the role of histone H1 as a DNA sequence-dependent architectural determinant of chromatin structure and of transcriptional activity in chromatin. The Xenopus laevis oocyte- and somatic-type 5S rRNA genes are differentially transcribed in embryonic chromosomes in vivo depending on the incorporation of somatic histone H1 into chromatin. We establish that this effect can be reconstructed at the level of a single nucleosome. H1 selectively represses oocyte-type 5S rRNA genes by directing the stable positioning of a nucleosome such that transcription factors cannot bind to the gene. This effect does not occur on the somatic-type genes. Histone H1 binds to the 5' end of the nucleosome core on the somatic 5S rRNA gene, leaving key regulatory elements in the promoter accessible, while histone H1 binds to the 3' end of the nucleosome core on the oocyte 5S rRNA genes, specifically blocking access to a key promoter element (the C box). TFIIIA can bind to the somatic 5S rRNA gene assembled into a nucleosome in the presence of H1. Because H1 binds with equivalent affinities to nucleosomes containing either gene, we establish that it is the sequence-selective assembly of a specific repressive chromatin structure on the oocyte 5S rRNA genes that accounts for differential transcriptional repression. Thus, general components of chromatin can determine the assembly of specific regulatory nucleoprotein complexes.
Figures
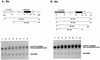
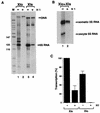
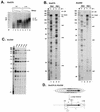
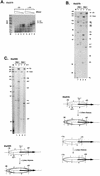
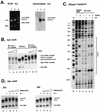
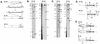
Similar articles
-
Specific regulation of Xenopus chromosomal 5S rRNA gene transcription in vivo by histone H1.Genes Dev. 1994 May 15;8(10):1147-59. doi: 10.1101/gad.8.10.1147. Genes Dev. 1994. PMID: 7926720
-
Differential nucleosome positioning on Xenopus oocyte and somatic 5 S RNA genes determines both TFIIIA and H1 binding: a mechanism for selective H1 repression.J Mol Biol. 1998 Sep 25;282(3):683-97. doi: 10.1006/jmbi.1998.2087. J Mol Biol. 1998. PMID: 9737930
-
Chromosomal organization of Xenopus laevis oocyte and somatic 5S rRNA genes in vivo.Mol Cell Biol. 1992 Jan;12(1):45-55. doi: 10.1128/mcb.12.1.45-55.1992. Mol Cell Biol. 1992. PMID: 1729615 Free PMC article.
-
The role of histone H1 in chromatin condensation and transcriptional repression.Genetica. 1999;106(1-2):117-24. doi: 10.1023/a:1003745315540. Genetica. 1999. PMID: 10710717 Review.
-
Xenopus transcription factor IIIA and the 5S nucleosome: development of a useful in vitro system.Biochem Cell Biol. 2003 Jun;81(3):177-84. doi: 10.1139/o03-043. Biochem Cell Biol. 2003. PMID: 12897852 Review.
Cited by
-
Chromosomal protein HMGN1 enhances the acetylation of lysine 14 in histone H3.EMBO J. 2005 Sep 7;24(17):3038-48. doi: 10.1038/sj.emboj.7600768. Epub 2005 Aug 11. EMBO J. 2005. PMID: 16096646 Free PMC article.
-
Global gene expression analysis reveals specific and redundant roles for H1 variants, H1c and H1(0), in gene expression regulation.Gene. 2008 May 15;414(1-2):10-8. doi: 10.1016/j.gene.2008.01.025. Epub 2008 Feb 16. Gene. 2008. PMID: 18372120 Free PMC article.
-
Specific binding of high-mobility-group I (HMGI) protein and histone H1 to the upstream AT-rich region of the murine beta interferon promoter: HMGI protein acts as a potential antirepressor of the promoter.Mol Cell Biol. 1999 Apr;19(4):2803-16. doi: 10.1128/MCB.19.4.2803. Mol Cell Biol. 1999. PMID: 10082546 Free PMC article.
-
Oocyte-specific linker histone H1foo is an epigenomic modulator that decondenses chromatin and impairs pluripotency.Epigenetics. 2012 Sep;7(9):1029-36. doi: 10.4161/epi.21492. Epub 2012 Aug 7. Epigenetics. 2012. PMID: 22868987 Free PMC article.
-
Reconstitution of enhancer function in paternal pronuclei of one-cell mouse embryos.Mol Cell Biol. 2001 Aug;21(16):5531-40. doi: 10.1128/MCB.21.16.5531-5540.2001. Mol Cell Biol. 2001. PMID: 11463835 Free PMC article.
References
-
- Allan J, Hartman P G, Crane-Robinson C, Aviles F X. The structure of histone H1 and its location in chromatin. Nature. 1980;288:675–679. - PubMed
-
- Andrews M T, Brown D D. Transient activation of oocyte 5S RNA genes in Xenopus embryos by raising the level of the trans-acting factor TFIIIA. Cell. 1987;51:445–453. - PubMed
-
- Bazett-Jones D P, LeBlanc B, Herfort M, Moss T. Short range DNA looping by the Xenopus HMG-box transcription factor, xUBF. Science. 1994;264:1134–1136. - PubMed
-
- Becker P B. The establishment of active promoters in chromatin. Bioessays. 1994;16:541–547. - PubMed
MeSH terms
Substances
LinkOut - more resources
Full Text Sources