Regulation of endothelial cell motility by complexes of tetraspan molecules CD81/TAPA-1 and CD151/PETA-3 with alpha3 beta1 integrin localized at endothelial lateral junctions
- PMID: 9566977
- PMCID: PMC2132738
- DOI: 10.1083/jcb.141.3.791
Regulation of endothelial cell motility by complexes of tetraspan molecules CD81/TAPA-1 and CD151/PETA-3 with alpha3 beta1 integrin localized at endothelial lateral junctions
Abstract
Cell-to-cell junction structures play a key role in cell growth rate control and cell polarization. In endothelial cells (EC), these structures are also involved in regulation of vascular permeability and leukocyte extravasation. To identify novel components in EC intercellular junctions, mAbs against these cells were produced and selected using a morphological screening by immunofluorescence microscopy. Two novel mAbs, LIA1/1 and VJ1/16, specifically recognized a 25-kD protein that was selectively localized at cell-cell junctions of EC, both in the primary formation of cell monolayers and when EC reorganized in the process of wound healing. This antigen corresponded to the recently cloned platelet-endothelial tetraspan antigen CD151/PETA-3 (platelet-endothelial tetraspan antigen-3), and was consistently detected at EC cell-cell contact sites. In addition to CD151/PETA-3, two other members of the tetraspan superfamily, CD9 and CD81/ TAPA-1 (target of antiproliferative antibody-1), localized at endothelial cell-to-cell junctions. Biochemical analysis demonstrated molecular associations among tetraspan molecules themselves and those of CD151/ PETA-3 and CD9 with alpha3 beta1 integrin. Interestingly, mAbs directed to both CD151/PETA-3 and CD81/ TAPA-1 as well as mAb specific for alpha3 integrin, were able to inhibit the migration of ECs in the process of wound healing. The engagement of CD151/PETA-3 and CD81/TAPA-1 inhibited the movement of individual ECs, as determined by quantitative time-lapse video microscopy studies. Furthermore, mAbs against the CD151/PETA-3 molecule diminished the rate of EC invasion into collagen gels. In addition, these mAbs were able to increase the adhesion of EC to extracellular matrix proteins. Together these results indicate that CD81/TAPA-1 and CD151/PETA-3 tetraspan molecules are components of the endothelial lateral junctions implicated in the regulation of cell motility, either directly or by modulation of the function of the associated integrin heterodimers.
Figures
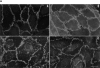
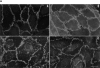
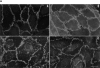
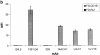
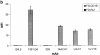
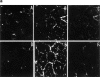
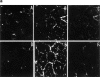
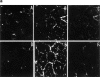
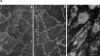
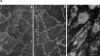
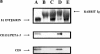
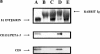
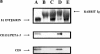
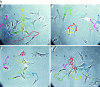
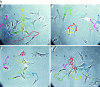
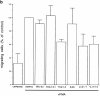
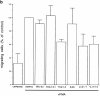
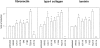
Similar articles
-
Selective tetraspan-integrin complexes (CD81/alpha4beta1, CD151/alpha3beta1, CD151/alpha6beta1) under conditions disrupting tetraspan interactions.Biochem J. 1999 May 15;340 ( Pt 1)(Pt 1):103-11. Biochem J. 1999. PMID: 10229664 Free PMC article.
-
PETA-3/CD151, a member of the transmembrane 4 superfamily, is localised to the plasma membrane and endocytic system of endothelial cells, associates with multiple integrins and modulates cell function.J Cell Sci. 1999 Mar;112 ( Pt 6):833-44. doi: 10.1242/jcs.112.6.833. J Cell Sci. 1999. PMID: 10036233
-
Tetraspanins are localized at motility-related structures and involved in normal human keratinocyte wound healing migration.J Invest Dermatol. 2000 Jun;114(6):1126-35. doi: 10.1046/j.1523-1747.2000.00998.x. J Invest Dermatol. 2000. PMID: 10844555
-
CD81 (TAPA-1): a molecule involved in signal transduction and cell adhesion in the immune system.Annu Rev Immunol. 1998;16:89-109. doi: 10.1146/annurev.immunol.16.1.89. Annu Rev Immunol. 1998. PMID: 9597125 Review.
-
Tetraspanin CD151 as a target for antibody-based cancer immunotherapy.Biochem Soc Trans. 2011 Apr;39(2):553-8. doi: 10.1042/BST0390553. Biochem Soc Trans. 2011. PMID: 21428938 Review.
Cited by
-
Functional implications of tetraspanin proteins in cancer biology.Cancer Sci. 2007 Nov;98(11):1666-77. doi: 10.1111/j.1349-7006.2007.00584.x. Epub 2007 Aug 24. Cancer Sci. 2007. PMID: 17727684 Free PMC article. Review.
-
HLA ligand profiles of primary renal cell carcinoma maintained in metastases.Cancer Immunol Immunother. 2009 Sep;58(9):1407-17. doi: 10.1007/s00262-008-0655-6. Epub 2009 Feb 1. Cancer Immunol Immunother. 2009. PMID: 19184600 Free PMC article.
-
Molecular mechanisms involved in vascular interactions of the Lyme disease pathogen in a living host.PLoS Pathog. 2008 Oct 3;4(10):e1000169. doi: 10.1371/journal.ppat.1000169. PLoS Pathog. 2008. PMID: 18833295 Free PMC article.
-
Immune Targeting of Tetraspanins Involved in Cell Invasion and Metastasis.Front Immunol. 2018 Jun 12;9:1277. doi: 10.3389/fimmu.2018.01277. eCollection 2018. Front Immunol. 2018. PMID: 29946318 Free PMC article. Review.
-
Expression of CD151 in human atherosclerotic artery and its implication.J Huazhong Univ Sci Technolog Med Sci. 2005;25(6):629-31. doi: 10.1007/BF02896154. J Huazhong Univ Sci Technolog Med Sci. 2005. PMID: 16696309
References
-
- Ades EW, Candal FJ, Swerlick RA, George VG, Summers S, Bosse DC, Lawley TJ. HMEC-1: Establishment of an immortalized human microvascular endothelial cell line. J Invest Dermatol. 1992;99:683–690. - PubMed
-
- Anderson JM, Balda MS, Fanning AS. The structure and regulation of tight junctions. Curr Opin Cell Biol. 1993;5:772–778. - PubMed
-
- Angelisová P, Hilgert I, Horejsi V. Association of four antigens of the tetraspan family (CD37, CD53, TAPA-1, and R2/C33) with MHC class II glycoproteins. Immunogenetics. 1994;39:249–256. - PubMed
-
- Ashman, L.K., S. Fitter, P.M. Sincock, L. Nguyen, and A.C. Cambareri. 1997. Summary report: CD151. In Leucocyte Typing VI. Ed by T. Kishimoto, M. Miyasaka, D. Mason, K. Sugamura, T. Springer, S. Shaw, S.M. Goyert, L. Moretta, H. Zola, A.E.-G.Kr. von dem Borne, K. Okumura, and K. Kikutani. Garland Publishing, New York.
Publication types
MeSH terms
Substances
LinkOut - more resources
Full Text Sources
Other Literature Sources
Research Materials