Vinculin proteolysis unmasks an ActA homolog for actin-based Shigella motility
- PMID: 9298981
- PMCID: PMC2132544
- DOI: 10.1083/jcb.138.6.1255
Vinculin proteolysis unmasks an ActA homolog for actin-based Shigella motility
Abstract
To generate the forces needed for motility, the plasma membranes of nonmuscle cells adopt an activated state that dynamically reorganizes the actin cytoskeleton. By usurping components from focal contacts and the actin cytoskeleton, the intracellular pathogens Shigella flexneri and Listeria monocytogenes use molecular mimicry to create their own actin-based motors. We raised an antibody (designated FS-1) against the FEFPPPPTDE sequence of Listeria ActA, and this antibody: (a) localized at the trailing end of motile intracellular Shigella, (b) inhibited intracellular locomotion upon microinjection of Shigella-infected cells, and (c) cross-reacted with the proteolytically derived 90-kD human vinculin head fragment that contains the Vinc-1 oligoproline sequence, PDFPPPPPDL. Antibody FS-1 reacted only weakly with full-length vinculin, suggesting that the Vinc-1 sequence in full-length vinculin may be masked by its tail region and that this sequence is unmasked by proteolysis. Immunofluoresence staining with a monoclonal antibody against the head region of vinculin (Vin 11-5) localized to the back of motile bacteria (an identical staining pattern observed with the anti-ActA FS-1 antibody), indicating that motile bacteria attract a form of vinculin containing an unmasked Vinc-1 oligoproline sequence. Microinjection of submicromolar concentrations of a synthetic Vinc-1 peptide arrested Shigella intracellular motility, underscoring the functional importance of this sequence. Western blots revealed that Shigella infection induces vinculin proteolysis in PtK2 cells and generates p90 head fragment over the same 1-3 h time frame when intracellular bacteria move within the host cell cytoplasm. We also discovered that microinjected p90, but not full-length vinculin, accelerates rates of pathogen motility by a factor of 3 +/- 0.4 in Shigella-infected PtK2 cells. These experiments suggest that vinculin p90 is a rate-limiting component in actin-based Shigella motility, and that supplementing cells with p90 stimulates rocket tail growth. Earlier findings demonstrated that vinculin p90 binds to IcsA (Suzuki, T.A., S. Saga, and C. Sasakawa. 1996. J. Biol. Chem. 271:21878-21885) and to vasodilator-stimulated phosphoprotein (VASP) (Brindle, N.P.J., M. R. Hold, J.E. Davies, C.J. Price, and D.R. Critchley. 1996. Biochem. J. 318:753-757). We now offer a working model in which proteolysis unmasks vinculin's ActA-like oligoproline sequence. Unmasking of this site serves as a molecular switch that initiates assembly of an actin-based motility complex containing VASP and profilin.
Figures
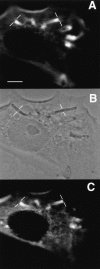
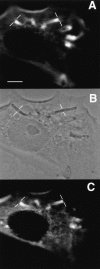
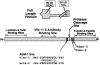
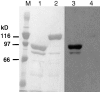
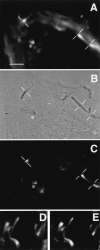
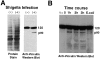
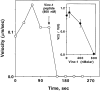
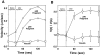
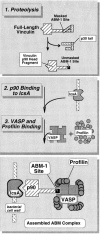
Similar articles
-
Recognition of two classes of oligoproline sequences in profilin-mediated acceleration of actin-based Shigella motility.J Cell Biol. 1996 Apr;133(1):49-59. doi: 10.1083/jcb.133.1.49. J Cell Biol. 1996. PMID: 8601612 Free PMC article.
-
Shigella interactions with the actin cytoskeleton in the absence of Ena/VASP family proteins.Cell Microbiol. 2004 Apr;6(4):355-66. doi: 10.1046/j.1462-5822.2003.00359.x. Cell Microbiol. 2004. PMID: 15009027
-
Shigella actin-based motility in the absence of vinculin.Cell Motil Cytoskeleton. 1997;37(1):44-53. doi: 10.1002/(SICI)1097-0169(1997)37:1<44::AID-CM5>3.0.CO;2-H. Cell Motil Cytoskeleton. 1997. PMID: 9142438
-
The focal adhesion phosphoprotein, VASP.Int J Biochem Cell Biol. 1998 Mar;30(3):307-11. doi: 10.1016/s1357-2725(97)00101-5. Int J Biochem Cell Biol. 1998. PMID: 9611773 Review.
-
Actin-based bacterial motility.Curr Opin Cell Biol. 1995 Feb;7(1):94-101. doi: 10.1016/0955-0674(95)80050-6. Curr Opin Cell Biol. 1995. PMID: 7755995 Review.
Cited by
-
Molecular basis of the intracellular spreading of Shigella.Infect Immun. 2001 Oct;69(10):5959-66. doi: 10.1128/IAI.69.10.5959-5966.2001. Infect Immun. 2001. PMID: 11553531 Free PMC article. Review. No abstract available.
-
Vaccinia locomotion in host cells: evidence for the universal involvement of actin-based motility sequences ABM-1 and ABM-2.Proc Natl Acad Sci U S A. 1998 Nov 10;95(23):13917-22. doi: 10.1073/pnas.95.23.13917. Proc Natl Acad Sci U S A. 1998. PMID: 9811901 Free PMC article.
-
Actin-based motility of intracellular microbial pathogens.Microbiol Mol Biol Rev. 2001 Dec;65(4):595-626, table of contents. doi: 10.1128/MMBR.65.4.595-626.2001. Microbiol Mol Biol Rev. 2001. PMID: 11729265 Free PMC article. Review.
-
Gelsolin mediates calcium-dependent disassembly of Listeria actin tails.Proc Natl Acad Sci U S A. 2005 Feb 8;102(6):1921-6. doi: 10.1073/pnas.0409062102. Epub 2005 Jan 25. Proc Natl Acad Sci U S A. 2005. PMID: 15671163 Free PMC article.
-
Dynamics of actin-based movement by Rickettsia rickettsii in vero cells.Infect Immun. 1999 Aug;67(8):4201-7. doi: 10.1128/IAI.67.8.4201-4207.1999. Infect Immun. 1999. PMID: 10417192 Free PMC article.
References
-
- Chakraborty T, Ebel F, Domann E, Niebuhr K, Gerstel B, Pistor S, Temm-Grove J, Jockusch BM, Reinhard M, Walter U, et al. A focal adhesion factor directly linking intracellularly motile Listeria monocytogenes and Listeria ivanoviito the actin-based cytoskeleton of mammalian cells. EMBO (Eur Mol Biol Organ) J. 1995;14:1314–1321. - PMC - PubMed
-
- Cudmore S, Cossart P, Griffiths G, Way M. Actin-based motility of vaccinia virus. Nature (Lond) 1995;378:636–638. - PubMed
Publication types
MeSH terms
Substances
Grants and funding
LinkOut - more resources
Full Text Sources
Other Literature Sources
Molecular Biology Databases
Miscellaneous