Regulation of actin polymerization in cell-free systems by GTPgammaS and Cdc42
- PMID: 9230078
- PMCID: PMC2138194
- DOI: 10.1083/jcb.138.2.363
Regulation of actin polymerization in cell-free systems by GTPgammaS and Cdc42
Abstract
We have established a cell-free system to investigate pathways that regulate actin polymerization. Addition of GTPgammaS to lysates of polymorphonuclear leukocytes (PMNs) or Dictyostelium discoideum amoeba induced formation of filamentous actin. The GTPgammaS appeared to act via a small G-protein, since it was active in lysates ofD. discoideum mutants missing either the alpha2- or beta-subunit of the heterotrimeric G-protein required for chemoattractant-induced actin polymerization in living cells. Furthermore, recombinant Cdc42, but not Rho or Rac, induced polymerization in the cell-free system. The Cdc42-induced increase in filamentous actin required GTPgammaS binding and was inhibited by a fragment of the enzyme PAK1 that binds Cdc42. In a high speed supernatant, GTPgammaS alone was ineffective, but GTPgammaS-loaded Cdc42 induced actin polymerization, suggesting that the response was limited by guanine nucleotide exchange. Stimulating exchange by chelating magnesium, by adding acidic phospholipids, or by adding the exchange factors Cdc24 or Dbl restored the ability of GTPgammaS to induce polymerization. The stimulation of actin polymerization did not correlate with PIP2 synthesis.
Figures
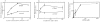
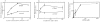
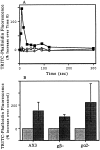
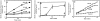
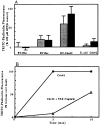
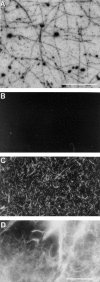
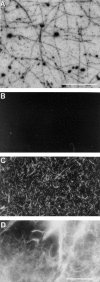
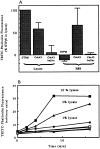
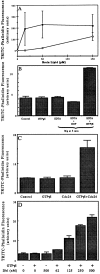
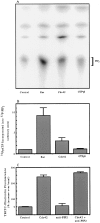
Similar articles
-
Corequirement of specific phosphoinositides and small GTP-binding protein Cdc42 in inducing actin assembly in Xenopus egg extracts.J Cell Biol. 1998 Mar 9;140(5):1125-36. doi: 10.1083/jcb.140.5.1125. J Cell Biol. 1998. PMID: 9490725 Free PMC article.
-
GTPgammaS-induced actin polymerisation in vitro: ATP- and phosphoinositide-independent signalling via Rho-family proteins and a plasma membrane-associated guanine nucleotide exchange factor.J Cell Sci. 1998 Jun;111 ( Pt 11):1583-94. doi: 10.1242/jcs.111.11.1583. J Cell Sci. 1998. PMID: 9580566
-
Mechanism of Cdc42-induced actin polymerization in neutrophil extracts.J Cell Biol. 1998 Aug 24;142(4):1001-12. doi: 10.1083/jcb.142.4.1001. J Cell Biol. 1998. PMID: 9722612 Free PMC article.
-
Regulation of phosphorylation pathways by p21 GTPases. The p21 Ras-related Rho subfamily and its role in phosphorylation signalling pathways.Eur J Biochem. 1996 Dec 1;242(2):171-85. doi: 10.1111/j.1432-1033.1996.0171r.x. Eur J Biochem. 1996. PMID: 8973630 Review.
-
p21-activated protein kinase: a crucial component of morphological signaling?Trends Biochem Sci. 1999 Sep;24(9):350-5. doi: 10.1016/s0968-0004(99)01442-5. Trends Biochem Sci. 1999. PMID: 10470034 Review.
Cited by
-
WASP-interacting protein is important for actin filament elongation and prompt pseudopod formation in response to a dynamic chemoattractant gradient.Mol Biol Cell. 2006 Oct;17(10):4564-75. doi: 10.1091/mbc.e05-10-0994. Epub 2006 Aug 9. Mol Biol Cell. 2006. PMID: 16899512 Free PMC article.
-
Screening of a PKC zeta-specific kinase inhibitor PKCzI257.3 which inhibits EGF-induced breast cancer cell chemotaxis.Invest New Drugs. 2010 Jun;28(3):268-75. doi: 10.1007/s10637-009-9242-8. Epub 2009 Mar 27. Invest New Drugs. 2010. PMID: 19326049
-
Activation by Cdc42 and PIP(2) of Wiskott-Aldrich syndrome protein (WASp) stimulates actin nucleation by Arp2/3 complex.J Cell Biol. 2000 Sep 18;150(6):1311-20. doi: 10.1083/jcb.150.6.1311. J Cell Biol. 2000. PMID: 10995437 Free PMC article.
-
Corequirement of specific phosphoinositides and small GTP-binding protein Cdc42 in inducing actin assembly in Xenopus egg extracts.J Cell Biol. 1998 Mar 9;140(5):1125-36. doi: 10.1083/jcb.140.5.1125. J Cell Biol. 1998. PMID: 9490725 Free PMC article.
-
Regulation of protein transport from the Golgi complex to the endoplasmic reticulum by CDC42 and N-WASP.Mol Biol Cell. 2002 Mar;13(3):866-79. doi: 10.1091/mbc.01-12-0579. Mol Biol Cell. 2002. PMID: 11907268 Free PMC article.
References
-
- Bokoch GMS. Regulation of the human neutrophil NADPH oxidase by the Rac GTP-binding proteins. Curr Opin Cell Biol. 1994;6:212–218. - PubMed
-
- Bokoch GM, Bohl BP, Chuang T-H. Guanine nucleotide exchange regulates membrane translocation of Rac/Rho GTP-binding proteins. J Biol Chem. 1994;269:31674–31679. - PubMed
Publication types
MeSH terms
Substances
Grants and funding
LinkOut - more resources
Full Text Sources
Research Materials
Miscellaneous