Expression of normal and mutant huntingtin in the developing brain
- PMID: 8757264
- PMCID: PMC6578889
- DOI: 10.1523/JNEUROSCI.16-17-05523.1996
Expression of normal and mutant huntingtin in the developing brain
Abstract
Huntington's disease (HD) is caused by a genetic mutation that results in a polyglutamine expansion in huntingtin. The time course of neuronal loss in the HD striatum and other affected brain regions before the onset of symptoms is unknown. To determine the potential influence of huntingtin on brain development, we examined its expression in the developing mouse and in human control and HD brain. By Western blot, huntingtin was detected throughout the adult mouse brain and at all stages of embryonic and postnatal brain development. The protein increased significantly between postnatal day 7 (P7) and P15, which marks a period of active neuronal differentiation and enhanced sensitivity to excitotoxic injury in the rodent striatum. Immunoreactivity was found in neurons throughout the brain and localized mostly to the somatodendritic cytoplasm and to axons in fiber bundles. Staining was variable in different groups of neurons and within the same cell population. In developing brain, huntingtin was limited primarily to neuronal perikarya. Increased immunoreactivity in large neurons followed the gradient of neurogenesis and appeared in the basal forebrain and brainstem by embryonic days 15-17, in regions of cortex by P0-P1, and in the striatum by P7. In human brain at midgestation (19-21 weeks), huntingtin was detected in all regions. The brain of a 10-week-old infant with the expanded HD allele expressed a higher molecular weight mutant form of huntingtin at levels comparable to those of the wild-type protein. Thus, mutant huntingtin is expressed before neuronal maturation is complete. Results suggest that huntingtin has an important constitutive role in neurons during brain development, that heterogeneity in neuronal expression of the protein is developmentally regulated, and that the intraneuronal distribution of huntingtin increases in parallel with neuronal maturation. The presence of mutant huntingtin in the immature HD brain raises the possibility that neurons may be affected during brain development and possibly in the postnatal period when vulnerability to excitotoxic injury is at its peak.
Figures
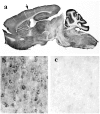
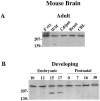
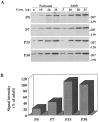
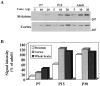
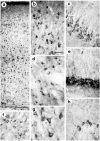
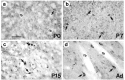
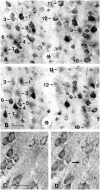
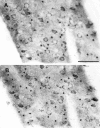
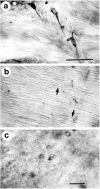
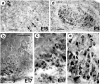
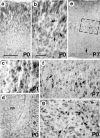
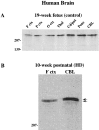
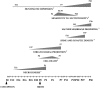
Similar articles
-
Transgenic mice expressing mutated full-length HD cDNA: a paradigm for locomotor changes and selective neuronal loss in Huntington's disease.Philos Trans R Soc Lond B Biol Sci. 1999 Jun 29;354(1386):1035-45. doi: 10.1098/rstb.1999.0456. Philos Trans R Soc Lond B Biol Sci. 1999. PMID: 10434303 Free PMC article.
-
Huntingtin localization in brains of normal and Huntington's disease patients.Ann Neurol. 1997 Oct;42(4):604-12. doi: 10.1002/ana.410420411. Ann Neurol. 1997. PMID: 9382472
-
Wild-type and mutant huntingtins function in vesicle trafficking in the secretory and endocytic pathways.Exp Neurol. 1998 Jul;152(1):34-40. doi: 10.1006/exnr.1998.6832. Exp Neurol. 1998. PMID: 9682010
-
[Huntington disease. A review].Invest Clin. 2000 Jun;41(2):117-41. Invest Clin. 2000. PMID: 10961047 Review. Spanish.
-
Selective degeneration in YAC mouse models of Huntington disease.Brain Res Bull. 2007 Apr 30;72(2-3):124-31. doi: 10.1016/j.brainresbull.2006.10.018. Epub 2006 Nov 16. Brain Res Bull. 2007. PMID: 17352936 Review.
Cited by
-
Wild-type huntingtin plays a role in brain development and neuronal survival.Mol Neurobiol. 2003 Dec;28(3):259-76. doi: 10.1385/MN:28:3:259. Mol Neurobiol. 2003. PMID: 14709789 Review.
-
Functional imaging in Huntington's disease.Exp Neurol. 2009 Apr;216(2):272-7. doi: 10.1016/j.expneurol.2008.12.015. Epub 2009 Jan 3. Exp Neurol. 2009. PMID: 19171138 Free PMC article. Review.
-
Cellular localization of huntingtin in striatal and cortical neurons in rats: lack of correlation with neuronal vulnerability in Huntington's disease.J Neurosci. 1999 Feb 15;19(4):1189-202. doi: 10.1523/JNEUROSCI.19-04-01189.1999. J Neurosci. 1999. PMID: 9952397 Free PMC article.
-
TRIM37 is a primate-specific E3 ligase for Huntingtin and accounts for the striatal degeneration in Huntington's disease.Sci Adv. 2024 May 17;10(20):eadl2036. doi: 10.1126/sciadv.adl2036. Epub 2024 May 17. Sci Adv. 2024. PMID: 38758800 Free PMC article.
-
Abnormal Weight and Body Mass Index in Children with Juvenile Huntington's Disease.J Huntingtons Dis. 2015;4(3):231-238. doi: 10.3233/JHD-150152. J Huntingtons Dis. 2015. PMID: 26443925 Free PMC article.
References
-
- Albin R, Greenmayre JT. Alternative excitotoxic hypotheses. Neurology. 1992;42:733–738. - PubMed
-
- Albin RL, Reiner A, Anderson KD, Dure LS, IV, Handelin B, Balfour R, Whetsell WO, Jr, Penney JB, Young AB. Preferential loss of striato-external pallidal projection neurons in presymptomatic Huntington’s disease. Ann Neurol. 1992;31:425–430. - PubMed
-
- Andrew SE, Goldberg YP, Kremer B, Telenius H, Theilmann J, Adam S, Starr E, Squitieri F, Lin B, Kalchman MA, Graham RK, Hayden MR. The relationship between trinucleotide (CAG) repeat length and clinical features of Huntington’s disease. Nature Genet. 1993;4:398–403. - PubMed
-
- Aronin N, Chase K, Christine Y, Sapp E, Schwarz C, Matta N, Kornreich R, Landwehrmeyer B, Bird E, Beal MF, Vonsattel JP, Smith T, Carraway R, Boyce FM, Young AB, Penney JB, DiFiglia M. CAG expansion affects the expression of mutant huntingtin in the Huntington’s disease brain. Neuron. 1995;15:1193–1201. - PubMed
-
- Bayer SA. Neurogenesis in the rat neostriatum. Int J Dev Neurosci. 1984;2:163–175. - PubMed
Publication types
MeSH terms
Substances
Grants and funding
LinkOut - more resources
Full Text Sources
Other Literature Sources
Molecular Biology Databases