Nascent RNA cleavage by arrested RNA polymerase II does not require upstream translocation of the elongation complex on DNA
- PMID: 7503982
- PMCID: PMC3373964
Nascent RNA cleavage by arrested RNA polymerase II does not require upstream translocation of the elongation complex on DNA
Abstract
Obstacles incurred by RNA polymerase II during primary transcript synthesis have been identified in vivo and in vitro. Transcription past these impediments requires SII, an RNA polymerase II-binding protein. SII also activates a nuclease in arrested elongation complexes and this nascent RNA shortening precedes transcriptional readthrough. Here we show that in the presence of SII and nucleotides, transcript cleavage is detected during SII-dependent elongation but not during SII-independent transcription. Thus, under typical transcription conditions, SII is necessary but insufficient to activate RNA cleavage. RNA cleavage could serve to move RNA polymerase II away from the transcriptional impediment and/or permit RNA polymerase II multiple attempts at RNA elongation. By mapping the positions of the 3'-ends of RNAs and the elongation complex on DNA, we demonstrate that upstream movement of RNA polymerase II is not required for limited RNA shortening (seven to nine nucleotides) and reactivation of an arrested complex. Arrested complexes become elongation competent after removal of no more than nine nucleotides from the nascent RNA's 3'-end. Further cleavage of nascent RNA, however, does result in "backward" translocation of the enzyme. We also show that one round of RNA cleavage is insufficient for full readthrough at an arrest site, consistent with a previously suggested mechanism of SII action.
Figures
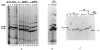
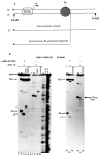
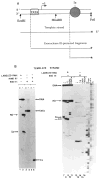
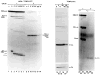
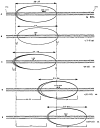
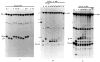
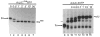
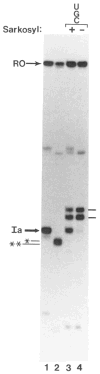
Similar articles
-
A DNA minor groove-binding ligand both potentiates and arrests transcription by RNA polymerase II. Elongation factor SII enables readthrough at arrest sites.J Mol Biol. 1994 Feb 25;236(3):725-37. doi: 10.1006/jmbi.1994.1185. J Mol Biol. 1994. PMID: 8114090
-
Transcription factors TFIIF, ELL, and Elongin negatively regulate SII-induced nascent transcript cleavage by non-arrested RNA polymerase II elongation intermediates.J Biol Chem. 2001 Jun 22;276(25):23109-14. doi: 10.1074/jbc.M101445200. Epub 2001 Mar 19. J Biol Chem. 2001. PMID: 11259417
-
The RNA polymerase II elongation complex. Factor-dependent transcription elongation involves nascent RNA cleavage.J Biol Chem. 1992 Aug 5;267(22):15516-22. J Biol Chem. 1992. PMID: 1379232 Free PMC article.
-
Transcription elongation factor SII.Bioessays. 2000 Apr;22(4):327-36. doi: 10.1002/(SICI)1521-1878(200004)22:4<327::AID-BIES3>3.0.CO;2-4. Bioessays. 2000. PMID: 10723030 Free PMC article. Review.
-
The transition from initiation to elongation by RNA polymerase II.Cold Spring Harb Symp Quant Biol. 1998;63:289-300. doi: 10.1101/sqb.1998.63.289. Cold Spring Harb Symp Quant Biol. 1998. PMID: 10384293 Review. No abstract available.
Cited by
-
Ccr4-Not and TFIIS Function Cooperatively To Rescue Arrested RNA Polymerase II.Mol Cell Biol. 2015 Jun 1;35(11):1915-25. doi: 10.1128/MCB.00044-15. Epub 2015 Mar 16. Mol Cell Biol. 2015. PMID: 25776559 Free PMC article.
-
Transcriptional pause, arrest and termination sites for RNA polymerase II in mammalian N- and c-myc genes.Nucleic Acids Res. 1999 Aug 1;27(15):3173-82. doi: 10.1093/nar/27.15.3173. Nucleic Acids Res. 1999. PMID: 10454615 Free PMC article.
-
Structural basis of transcription: alpha-amanitin-RNA polymerase II cocrystal at 2.8 A resolution.Proc Natl Acad Sci U S A. 2002 Feb 5;99(3):1218-22. doi: 10.1073/pnas.251664698. Epub 2002 Jan 22. Proc Natl Acad Sci U S A. 2002. PMID: 11805306 Free PMC article.
-
Mutations in the second largest subunit of RNA polymerase II cause 6-azauracil sensitivity in yeast and increased transcriptional arrest in vitro.J Biol Chem. 1996 Mar 22;271(12):6866-73. doi: 10.1074/jbc.271.12.6866. J Biol Chem. 1996. PMID: 8636112 Free PMC article.
-
Structural perspective on mutations affecting the function of multisubunit RNA polymerases.Microbiol Mol Biol Rev. 2006 Mar;70(1):12-36. doi: 10.1128/MMBR.70.1.12-36.2006. Microbiol Mol Biol Rev. 2006. PMID: 16524917 Free PMC article. Review.
References
Publication types
MeSH terms
Substances
Associated data
- Actions
Grants and funding
LinkOut - more resources
Full Text Sources
Other Literature Sources
Miscellaneous