Comparison of the amyloid plaque proteome in Down syndrome, early-onset Alzheimer's disease, and late-onset Alzheimer's disease
- PMID: 39825890
- PMCID: PMC11742868
- DOI: 10.1007/s00401-025-02844-z
Comparison of the amyloid plaque proteome in Down syndrome, early-onset Alzheimer's disease, and late-onset Alzheimer's disease
Abstract
Down syndrome (DS) is strongly associated with Alzheimer's disease (AD) due to APP overexpression, exhibiting Amyloid-β (Aβ) and Tau pathology similar to early-onset (EOAD) and late-onset AD (LOAD). We evaluated the Aβ plaque proteome of DS, EOAD, and LOAD using unbiased localized proteomics on post-mortem paraffin-embedded tissues from four cohorts (n = 20/group): DS (59.8 ± 4.99 y/o), EOAD (63 ± 4.07 y/o), LOAD (82.1 ± 6.37 y/o), and controls (66.4 ± 13.04). We identified differentially abundant proteins when comparing Aβ plaques and neighboring non-plaque tissue (FDR < 5%, fold-change > 1.5) in DS (n = 132), EOAD (n = 192), and LOAD (n = 128), with 43 plaque-associated proteins shared across all groups. Positive correlations were observed between plaque-associated proteins in DS and EOAD (R2 = .77), DS and LOAD (R2 = .73), and EOAD and LOAD (R2 = .67). Top gene ontology biological processes (GOBP) included lysosomal transport (p = 1.29 × 10-5) for DS, immune system regulation (p = 4.33 × 10-5) for EOAD, and lysosome organization (p = 0.029) for LOAD. Protein networks revealed a plaque-associated protein signature involving APP metabolism, immune response, and lysosomal functions. In DS, EOAD, and LOAD non-plaque vs. control tissue, we identified 263, 269, and 301 differentially abundant proteins, with 65 altered proteins shared across all cohorts. Non-plaque proteins in DS showed modest correlations with EOAD (R2 = .59) and LOAD (R2 = .33) compared to the correlation between EOAD and LOAD (R2 = .79). Top GOBP term for all groups was chromatin remodeling (p < 0.001), with additional terms for DS including extracellular matrix, and protein-DNA complexes and gene expression regulation for EOAD and LOAD. Our study reveals key functional characteristics of the amyloid plaque proteome in DS, compared to EOAD and LOAD, highlighting shared pathways in endo/lysosomal functions and immune responses. The non-plaque proteome revealed distinct alterations in ECM and chromatin structure, underscoring unique differences between DS and AD subtypes. Our findings enhance our understanding of AD pathogenesis and identify potential biomarkers and therapeutic targets.
Keywords: Alzheimer’s disease; Amyloid-β; Down syndrome; Neuropathology; Proteomics.
© 2025. The Author(s).
Conflict of interest statement
Declarations. Conflict of interest: J.F. reported receiving personal fees for service on the advisory boards, adjudication committees or speaker honoraria from AC Immune, Adamed, Alzheon, Biogen, Eisai, Esteve, Fujirebio, Ionis, Laboratorios Carnot, Life Molecular Imaging, Lilly, Lundbeck, Novo Nordisk, Perha, Roche, Zambón and outside the submitted work. J.F. reports holding a patent for markers of synaptopathy in neurodegenerative disease (licensed to ADx, EPI8382175.0).
Figures
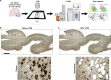
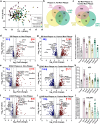
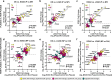
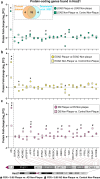
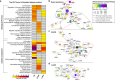
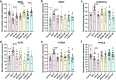
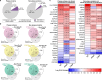
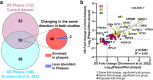
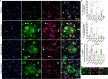
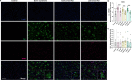
Update of
-
Comparison of the Amyloid Plaque Proteome in Down Syndrome, Early-Onset Alzheimer's Disease and Late-Onset Alzheimer's Disease.Res Sq [Preprint]. 2024 Jul 15:rs.3.rs-4469045. doi: 10.21203/rs.3.rs-4469045/v1. Res Sq. 2024. Update in: Acta Neuropathol. 2025 Jan 18;149(1):9. doi: 10.1007/s00401-025-02844-z PMID: 39070643 Free PMC article. Updated. Preprint.
Similar articles
-
Comparison of the Amyloid Plaque Proteome in Down Syndrome, Early-Onset Alzheimer's Disease and Late-Onset Alzheimer's Disease.Res Sq [Preprint]. 2024 Jul 15:rs.3.rs-4469045. doi: 10.21203/rs.3.rs-4469045/v1. Res Sq. 2024. Update in: Acta Neuropathol. 2025 Jan 18;149(1):9. doi: 10.1007/s00401-025-02844-z PMID: 39070643 Free PMC article. Updated. Preprint.
-
Amyloid-β peptide signature associated with cerebral amyloid angiopathy in familial Alzheimer's disease with APPdup and Down syndrome.Acta Neuropathol. 2024 Jul 18;148(1):8. doi: 10.1007/s00401-024-02756-4. Acta Neuropathol. 2024. PMID: 39026031 Free PMC article.
-
Patterns and severity of vascular amyloid in Alzheimer's disease associated with duplications and missense mutations in APP gene, Down syndrome and sporadic Alzheimer's disease.Acta Neuropathol. 2018 Oct;136(4):569-587. doi: 10.1007/s00401-018-1866-3. Epub 2018 May 16. Acta Neuropathol. 2018. PMID: 29770843 Free PMC article.
-
A systematic review of the efficacy and safety of anti-amyloid beta monoclonal antibodies in treatment of Alzheimer's disease.Expert Opin Biol Ther. 2024 Nov;24(11):1261-1269. doi: 10.1080/14712598.2024.2416947. Epub 2024 Nov 12. Expert Opin Biol Ther. 2024. PMID: 39432414 Review.
-
Impact of residual disease as a prognostic factor for survival in women with advanced epithelial ovarian cancer after primary surgery.Cochrane Database Syst Rev. 2022 Sep 26;9(9):CD015048. doi: 10.1002/14651858.CD015048.pub2. Cochrane Database Syst Rev. 2022. PMID: 36161421 Free PMC article. Review.
References
-
- Aladeokin AC, Akiyama T, Kimura A, Kimura Y, Takahashi-Jitsuki A, Nakamura H et al (2019) Network-guided analysis of hippocampal proteome identifies novel proteins that colocalize with Abeta in a mice model of early-stage Alzheimer’s disease. Neurobiol Dis. 10.1016/j.nbd.2019.104603 - PubMed
Publication types
MeSH terms
Substances
Grants and funding
LinkOut - more resources
Full Text Sources
Medical