RNA-dependent RNA polymerase of predominant human norovirus forms liquid-liquid phase condensates as viral replication factories
- PMID: 39705355
- PMCID: PMC11661447
- DOI: 10.1126/sciadv.adp9333
RNA-dependent RNA polymerase of predominant human norovirus forms liquid-liquid phase condensates as viral replication factories
Abstract
Many viral proteins form biomolecular condensates via liquid-liquid phase separation (LLPS) to support viral replication and evade host antiviral responses, and thus, they are potential targets for designing antivirals. In the case of nonenveloped positive-sense RNA viruses, forming such condensates for viral replication is unclear and less understood. Human noroviruses (HuNoVs) are positive-sense RNA viruses that cause epidemic and sporadic gastroenteritis worldwide. Here, we show that the RNA-dependent RNA polymerase (RdRp) of pandemic GII.4 HuNoV forms distinct condensates that exhibit all the signature properties of LLPS with sustained polymerase activity and the capability of recruiting components essential for viral replication. We show that such condensates are formed in HuNoV-infected human intestinal enteroid cultures and are the sites for genome replication. Our studies demonstrate the formation of phase-separated condensates as replication factories in a positive-sense RNA virus, which plausibly is an effective mechanism to dynamically isolate RdRp replicating the genomic RNA from interfering with the ribosomal translation of the same RNA.
Figures
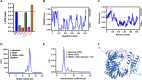
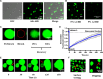
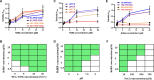
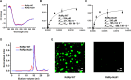
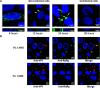
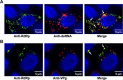
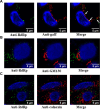
Update of
-
RNA-dependent RNA polymerase of predominant human norovirus forms liquid-liquid phase condensates as viral replication factories.bioRxiv [Preprint]. 2024 Sep 18:2023.08.24.554692. doi: 10.1101/2023.08.24.554692. bioRxiv. 2024. Update in: Sci Adv. 2024 Dec 20;10(51):eadp9333. doi: 10.1126/sciadv.adp9333 PMID: 39345611 Free PMC article. Updated. Preprint.
Similar articles
-
RNA-dependent RNA polymerase of predominant human norovirus forms liquid-liquid phase condensates as viral replication factories.bioRxiv [Preprint]. 2024 Sep 18:2023.08.24.554692. doi: 10.1101/2023.08.24.554692. bioRxiv. 2024. Update in: Sci Adv. 2024 Dec 20;10(51):eadp9333. doi: 10.1126/sciadv.adp9333 PMID: 39345611 Free PMC article. Updated. Preprint.
-
Bile acid-sensitive human norovirus strains are susceptible to sphingosine-1-phosphate receptor 2 inhibition.J Virol. 2024 Jul 23;98(7):e0202023. doi: 10.1128/jvi.02020-23. Epub 2024 Jun 17. J Virol. 2024. PMID: 38884472 Free PMC article.
-
Development of a broad-spectrum therapeutic Fc-nanobody for human noroviruses.J Virol. 2024 Jul 23;98(7):e0070724. doi: 10.1128/jvi.00707-24. Epub 2024 Jul 2. J Virol. 2024. PMID: 38953655 Free PMC article.
-
Depressing time: Waiting, melancholia, and the psychoanalytic practice of care.In: Kirtsoglou E, Simpson B, editors. The Time of Anthropology: Studies of Contemporary Chronopolitics. Abingdon: Routledge; 2020. Chapter 5. In: Kirtsoglou E, Simpson B, editors. The Time of Anthropology: Studies of Contemporary Chronopolitics. Abingdon: Routledge; 2020. Chapter 5. PMID: 36137063 Free Books & Documents. Review.
-
Impact of residual disease as a prognostic factor for survival in women with advanced epithelial ovarian cancer after primary surgery.Cochrane Database Syst Rev. 2022 Sep 26;9(9):CD015048. doi: 10.1002/14651858.CD015048.pub2. Cochrane Database Syst Rev. 2022. PMID: 36161421 Free PMC article. Review.
References
-
- Brangwynne C. P., Eckmann C. R., Courson D. S., Rybarska A., Hoege C., Gharakhani J., Julicher F., Hyman A. A., Germline P granules are liquid droplets that localize by controlled dissolution/condensation. Science 324, 1729–1732 (2009). - PubMed
-
- Zhang H., Ji X., Li P., Liu C., Lou J., Wang Z., Wen W., Xiao Y., Zhang M., Zhu X., Liquid-liquid phase separation in biology: Mechanisms, physiological functions and human diseases. Sci. China Life Sci. 63, 953–985 (2020). - PubMed
MeSH terms
Substances
Grants and funding
LinkOut - more resources
Full Text Sources
Medical
Research Materials