Human airway macrophages are metabolically reprogrammed by IFN-γ resulting in glycolysis-dependent functional plasticity
- PMID: 39620891
- PMCID: PMC11611294
- DOI: 10.7554/eLife.98449
Human airway macrophages are metabolically reprogrammed by IFN-γ resulting in glycolysis-dependent functional plasticity
Abstract
Airway macrophages (AM) are the predominant immune cell in the lung and play a crucial role in preventing infection, making them a target for host directed therapy. Macrophage effector functions are associated with cellular metabolism. A knowledge gap remains in understanding metabolic reprogramming and functional plasticity of distinct human macrophage subpopulations, especially in lung resident AM. We examined tissue-resident AM and monocyte-derived macrophages (MDM; as a model of blood derived macrophages) in their resting state and after priming with IFN-γ or IL-4 to model the Th1/Th2 axis in the lung. Human macrophages, regardless of origin, had a strong induction of glycolysis in response to IFN-γ or upon stimulation. IFN-γ significantly enhanced cellular energetics in both AM and MDM by upregulating both glycolysis and oxidative phosphorylation. Upon stimulation, AM do not decrease oxidative phosphorylation unlike MDM which shift to 'Warburg'-like metabolism. IFN-γ priming promoted cytokine secretion in AM. Blocking glycolysis with 2-deoxyglucose significantly reduced IFN-γ driven cytokine production in AM, indicating that IFN-γ induces functional plasticity in human AM, which is mechanistically mediated by glycolysis. Directly comparing responses between macrophages, AM were more responsive to IFN-γ priming and dependent on glycolysis for cytokine secretion than MDM. Interestingly, TNF production was under the control of glycolysis in AM and not in MDM. MDM exhibited glycolysis-dependent upregulation of HLA-DR and CD40, whereas IFN-γ upregulated HLA-DR and CD40 on AM independently of glycolysis. These data indicate that human AM are functionally plastic and respond to IFN-γ in a manner distinct from MDM. These data provide evidence that human AM are a tractable target for inhalable immunomodulatory therapies for respiratory diseases.
Keywords: Mycobacterium tuberculosis; airway macrophages; cytokines; human; immunology; inflammation; lipopolysaccharide; metabolism; polarization.
Plain language summary
Inside the human body, immune cells known as macrophages are constantly looking for microbes, cell debris and other potential threats to engulf and digest. If a macrophage detects a microbe, it activates and releases molecules called cytokines, which induce further immune responses that help to eliminate the invader. The macrophages found in the lungs, known as airway macrophages, defend against pollutants and airborne microbes and are therefore key for maintaining respiratory health. Despite this, previous studies have suggested that airway macrophages are not as good at responding to infections as other types of macrophages. Certain cytokines can cause macrophages to switch how they generate the chemical energy needed to fuel various processes in the cell. However, it remains unclear if it may be possible to develop therapies that boost airway macrophage activity during infection by modifying how they produce chemical energy. To investigate, Cox et al. compared how human airway macrophages and macrophages that originate in the blood alter their production of chemical energy in response to cues from the immune system that indicate an infection is present. The experiments showed that exposure to a specific cytokine known as IFN-γ caused both macrophage types to produce more chemical energy using a metabolic process known as glycolysis. Inhibiting glycolysis induced by IFN-γ had a much bigger effect on the ability of the airway macrophages to produce cytokines than it had on blood macrophages. Furthermore, glycolysis controlled the production of a particular cytokine called TNF in the airway macrophages, but not the blood macrophages. The findings demonstrate that airway macrophages alter how they produce chemical energy during infections in a different way to blood macrophages. Since TNF is a crucial cytokine for defending against respiratory infections, understanding how it is regulated in the lung could help researchers to develop inhalable therapies to boost its production in patients with respiratory infections that are difficult to treat. The specificity of this approach could ultimately limit side effects compared to therapies that act throughout the body.
© 2024, Cox et al.
Conflict of interest statement
DC, SC, CÓ, AB, OS, ED, KG, OÓ, DM, SO, FO, PN, JP, LG, SB, JK No competing interests declared
Figures
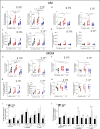
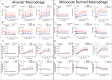
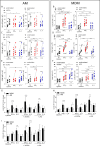
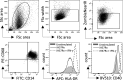
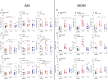
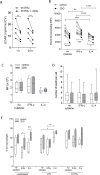
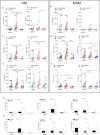
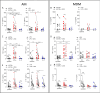
Update of
- doi: 10.1101/2024.03.20.585747
- doi: 10.7554/eLife.98449.1
- doi: 10.7554/eLife.98449.2
- doi: 10.7554/eLife.98449.3
Similar articles
-
Depressing time: Waiting, melancholia, and the psychoanalytic practice of care.In: Kirtsoglou E, Simpson B, editors. The Time of Anthropology: Studies of Contemporary Chronopolitics. Abingdon: Routledge; 2020. Chapter 5. In: Kirtsoglou E, Simpson B, editors. The Time of Anthropology: Studies of Contemporary Chronopolitics. Abingdon: Routledge; 2020. Chapter 5. PMID: 36137063 Free Books & Documents. Review.
-
Using Experience Sampling Methodology to Capture Disclosure Opportunities for Autistic Adults.Autism Adulthood. 2023 Dec 1;5(4):389-400. doi: 10.1089/aut.2022.0090. Epub 2023 Dec 12. Autism Adulthood. 2023. PMID: 38116059 Free PMC article.
-
Qualitative evidence synthesis informing our understanding of people's perceptions and experiences of targeted digital communication.Cochrane Database Syst Rev. 2019 Oct 23;10(10):ED000141. doi: 10.1002/14651858.ED000141. Cochrane Database Syst Rev. 2019. PMID: 31643081 Free PMC article.
-
Comparison of Two Modern Survival Prediction Tools, SORG-MLA and METSSS, in Patients With Symptomatic Long-bone Metastases Who Underwent Local Treatment With Surgery Followed by Radiotherapy and With Radiotherapy Alone.Clin Orthop Relat Res. 2024 Dec 1;482(12):2193-2208. doi: 10.1097/CORR.0000000000003185. Epub 2024 Jul 23. Clin Orthop Relat Res. 2024. PMID: 39051924
-
Trends in Surgical and Nonsurgical Aesthetic Procedures: A 14-Year Analysis of the International Society of Aesthetic Plastic Surgery-ISAPS.Aesthetic Plast Surg. 2024 Oct;48(20):4217-4227. doi: 10.1007/s00266-024-04260-2. Epub 2024 Aug 5. Aesthetic Plast Surg. 2024. PMID: 39103642 Review.
References
-
- Afkhami S, D’Agostino MR, Zhang A, Stacey HD, Marzok A, Kang A, Singh R, Bavananthasivam J, Ye G, Luo X, Wang F, Ang JC, Zganiacz A, Sankar U, Kazhdan N, Koenig JFE, Phelps A, Gameiro SF, Tang S, Jordana M, Wan Y, Mossman KL, Jeyanathan M, Gillgrass A, Medina MFC, Smaill F, Lichty BD, Miller MS, Xing Z. Respiratory mucosal delivery of next-generation COVID-19 vaccine provides robust protection against both ancestral and variant strains of SARS-CoV-2. Cell. 2022;185:896–915. doi: 10.1016/j.cell.2022.02.005. - DOI - PMC - PubMed
-
- Bharti R, Roy T, Verma S, Reddy DVS, Shafi H, Verma K, Raman SK, Pal S, Azmi L, Singh AK, Ray L, Mugale MN, Misra A. Transient, inhaled gene therapy with gamma interferon mitigates pathology induced by host response in a mouse model of tuberculosis. Tuberculosis. 2022;134:102198. doi: 10.1016/j.tube.2022.102198. - DOI - PubMed
-
- Bourigault M-L, Segueni N, Rose S, Court N, Vacher R, Vasseur V, Erard F, Le Bert M, Garcia I, Iwakura Y, Jacobs M, Ryffel B, Quesniaux VFJ. Relative contribution of IL-1α, IL-1β and TNF to the host response to Mycobacterium tuberculosis and attenuated M. bovis BCG. Immunity, Inflammation and Disease. 2013;1:47–62. doi: 10.1002/iid3.9. - DOI - PMC - PubMed
-
- Cahill C, O’Connell F, Gogan KM, Cox DJ, Basdeo SA, O’Sullivan J, Gordon SV, Keane J, Phelan JJ. The iron chelator desferrioxamine increases the efficacy of bedaquiline in primary human macrophages infected with BCG. International Journal of Molecular Sciences. 2021;22:2938. doi: 10.3390/ijms22062938. - DOI - PMC - PubMed
MeSH terms
Substances
Associated data
Grants and funding
LinkOut - more resources
Full Text Sources
Research Materials
Miscellaneous